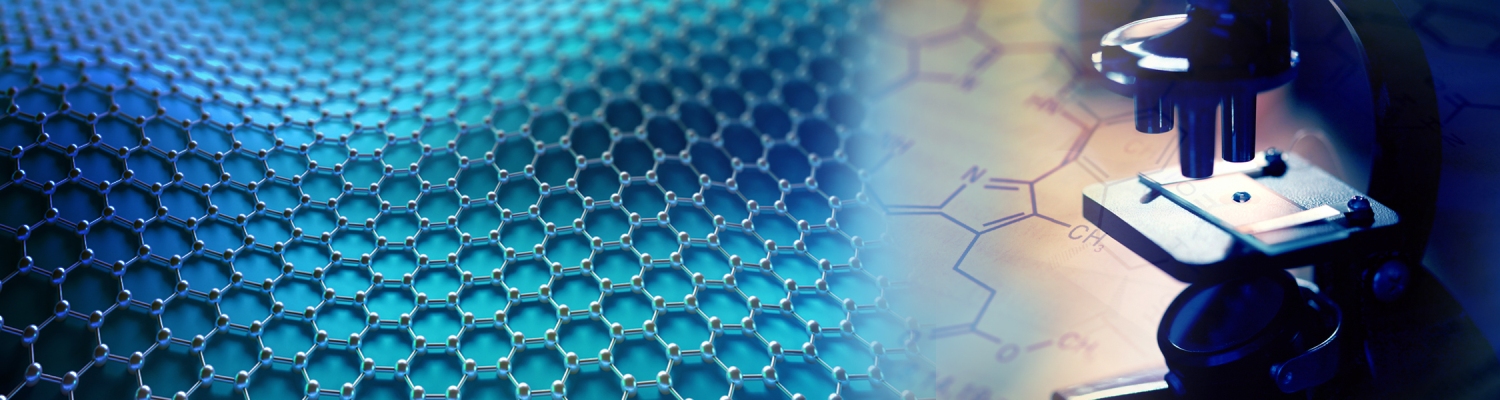
ISSN: 2641-6921
Tessy López*1,2, Verónica Sánchez de la Barquera3, Francisco J Padilla-Godínez1, Paola Ramírez1 and Esteban Gómez-López4
Received: October 03, 2020; Published:October 22, 2020
*Corresponding author: Tessy López, Department of Nanotechnology and Nanomedicine, Autonomous Metropolitan University Xochimilco, Coyoacán, Mexico City 04960, Mexico
DOI: 10.32474/MAMS.2020.03.000163
Objective: To describe patient outcomes with respect to the use of Cu/SiO2-TiO2 nanoparticles embedded in a polymeric gel as treatment for post-breast cancer chronic wounds with solid calcifications.
Methods: The nanoparticles were synthesized by the sol-gel method. The compound was incorporated into a polymeric gel matrix. SEM and TEM microscopy studies were carried out to evaluate grain size, morphology, and texture of the pure nanoparticles. The chronic wounds were treated by applying zinc oxide as emollient, Triticum vulgare (Italdermol®) and the Nano biocatalyst. The wound was covered with Solvaline® N dressings for the absorption of the exudate.
Results: The Cu/SiO2-TiO2 nanobiocatalyst displayed average particle size between 5 and 10 nm with high particle dispersion. Ordered arrangement of the nanoparticles was observed in TEM micrographs suggesting anatase crystalline structure. Microcalcifications extracted from the wound were suspected to be calcium carbonates and calcium oxalates, exhibiting sizes of the order of 20 μm. The administration of the nanoparticles with Triticum vulgare enhanced granulation tissue formation and revascularization by limiting bacterial infection. After 8 months of the first application the wound had completely healed in an atrophic scar, with no sign of inflammation or infection. No adverse effects were observed in the patient.
Conclusions: Post-breast cancer chronic wounds were successfully treated with Cu/SiO2-TiO2 nanoparticles. Good outcomes were observed in terms of tissue regeneration, wound healing, and infection hindering. Calcifications were eliminated. The nanoparticles can be used as a primary apposite to stimulate the autolytic debridement of injures due to their excellent local absorption and bactericide action.
Declaration of Interest: The study’s sponsors were not involved in the study design, the collection, analysis and interpretation of the data, the writing of this manuscript and the decision to submit this article for publication. The views express in this article are those of the authors.
Keywords: Cu/SiO2-TiO2, Chronic wounds, Breast cancer, Microcalcification, Tissue regeneration
Key Points:
a. A nanobiocatalyst based on mixed-oxides impregnated with transition metals was synthesized by the sol-gel method for the
treatment of a post-breast cancer chronic wound.
b. The nanobiocatalyst presented displayed average particle size between 5 and 10 nm with high particle dispersion and
ordered particle arrangement suggesting anatase crystalline structure as observed by SEM and TEM.
c. The post-breast cancer wound exhibited the formation of microcalcifications (20 μm) composed of calcium carbonates and
calcium oxalates.
d. The administration of the nanobiocatalyst enhanced the formation of granulation tissue and revascularization by limiting
bacterial infection.
e. The 30-years-old wound completely healed in an 8-months period, with no adverse effects detected.
f. The microcalcification formation was eliminated after tissue regeneration.
Despite recent advances in the detection and treatment of metastatic breast cancer, mortality related to this disease remains as one of the highest due to the emergence of therapy-resistant cancer cells [1-5]. In 2018, an estimated 2 million new global cases were diagnosed representing 23% of all cancers, incidence rates varying from 19.3 per 100,000 women in Eastern Africa to 89.7 per 100,000 women in Western Europe [6]. By 2030, the number of deaths in Latin America is expected to achieve 74,000 per year [7]. The Globocan 2018 estimated that in Mexico the incidence for breast cancer was 39.5 cases per 100,000 habitants, with a total of 27,283 new cases and 6,884 deaths [8,9]. Common treatments for nonmetastatic breast cancer include endocrine therapy and cytotoxic chemotherapy [10]. The main surgical approach for cancer of the breast in all stages, early or late, is a radical and total mastectomy, breast-conserving surgery, and breast reconstruction, all followed by irradiation [11]. Possible side effects of surgery include seroma, hematoma, lymphoedema, cording, restricted range of movement in the shoulder, nerve pain, tissue necrosis, microcalcification formation, and surgical site infection [12-15]. The incidence of wound complications after breast cancer surgery are substantial, ranging from 6 to 30% [16-19]. There are several factors related to increased risk of complications in post-surgical patients, including patient risk factors (e.g., diabetes, obesity, and smoking), [20,21] surgical technique, [22] and type of surgery. [23] In fact, it has been suggested in previous studies that radiation increases the risk of complications, both in patients receiving breast implants, [24-29] and in those treated with autologous reconstructions. Murthy et al. [30-32] proposed that delayed wound healing is associated with an increased rate of systemic recurrence after primary breast cancer excisional surgery, [33] therefore one main point of attention in postoperative care must be the control of wound-closure-hindering infections.
In past studies, we have evaluated the healing properties of copper nanoparticles stabilized in a titania-silica mixed oxide matrix (Cu/SiO2-TiO2) as a treatment for chronic diabetic foot ulcers [34,35]. The nanoparticles prepared by the sol-gel process allowed to avoid a programmed supracondylar amputation in the patient, with favorable clinic evolution of the chronic ulcer by enhancing tissue regeneration and wound healing.
The aim of this study was to determine the wound healing properties of the Cu/SiO2-TiO2 nanoparticles for the management of breast-cancer-related chronic wounds in combination with Triticum vulgare (used as a treatment of oral mucositis by radiotherapy and chemotherapy in cancer patients) [36] and Solvaline® N (wound dressing).
Ethanol (99.8%), copper acetylacetone (97%), sulfuric acid (98%), phosphoric acid (98%), tetraethyl orthosilicate (98%) and titanium butoxide (98%) were purchased from Sigma Aldrich Company (Saint-Louis, MO, USA). Solvaline® dressings were purchased from Lohmann & Rauscher (Milwaukee, WI, USA), and aqueous extract of Triticum vulgare (Italdermol®) was obtained from Italmex (Mexico City, CDMX, Mexico).
The nanoparticles were synthesized following the procedure of secrecy No. 101/100/014/13. A flask with deionized water and ethanol was kept at room temperature and constant stirring, the adequate amount of copper acetylacetonate was added to this mixture, then the pH was adjusted to pH 1 with sulfuric and phosphoric acids. During a period of 4h, tetraethyl orthosilicate and titanium butoxide were added dropwise to the mixture under stirring. Once the alkoxides were completely added, the temperature was increased while stirring until gelation. The nanoparticles were obtained when the gel dried. The compound was incorporated into a polymeric gel matrix.
The grain size, morphology, and texture of the pure nanoparticles with no polymeric gel matrix were characterized by scanning electron microscopy (SEM) in a JEOL JSM-6010LV microscope equipped with an energy dispersive spectroscopic (EDS) microanalysis system (OXFORD). The nanoparticles’ size was determined by transmission electron microscopy (TEM) in a JEOL JEM-2100F, operated at 120 kV voltage. The images were obtained using a CCD Mega Vision (III) camera.
A 78-years-old female patient from Mexico City presents a family history of diabetes mellitus and hypertension, as well as cases of chronic venous insufficiency and deep vein thrombosis, from both mother and father lineages. She presents type-2 diabetes mellitus for 15 years treated with biguanide and insulin 30-0-20, with medication non-adherence problems. The patient refers previous history of mixed dyslipidemia and hypertension. Furthermore, the patient was diagnosed with Invasive Ductal Carcinoma (IDC) in the left breast thirty years ago, which conducted to total mastectomy and irradiation. The breast cancer exhibited total remission for 30 years. The patient reported to medical consultation for presenting small wounds in the chest and secondary calcifications that were growing inside and outside the injury since the beginning of irradiation therapy. During first physical exploration blood pressure was 140/90 mmHg, heart frequency had a rate of 76 beats per minute, respiratory rate of 18 breath per minute, temperature was 36.9°C. The patient denies fever, general discomfort, asthenia, and adynamia. The size of the wound located in the left thorax is 2.0 x 1.0 x 1.5 cm with macerated borders (Figure 1a&1b), the perilesional skin showed edema, erythema, and hyperpigmentation, with yellow exudate and foul odorous suppuration, visible calcifications were identified inside the wound. She exhibits a scar due to the previous total mastectomy of the left breast. The patient refers moderate pain at palpation and during wound cleaning.
Figure 1: Post-breast cancer chronic wound with solid calcifications. The size of the wound is 2.0 x 1.0 x 1.5 cm with macerated borders. Calcifications are identified with black arrows.
Chronic radiation-induced ulcer in the chest wall after surgery in breast cancer. Risk factors for systemic recurrence: female, total mastectomy, chronic untreated ulcers, type-2 diabetes mellitus, hypertension.
Advanced wound care was initiated by applying zinc oxide (ZnO) on the macerated skin due to its epithelialization and infection-control properties [37]. The Cu/SiO2-TiO2 nanoparticles embedded in a polymeric matrix were applied in combination with Triticum vulgare inside the wounds to generate granulation tissue and limit bacterial infection. The wound was covered with Solvaline® N dressings for the absorption of the exudate. Additional management included prophylaxis antibiotics on one occasion and analgesics each 12 hours when pain was presented.
SEM images showed Cu/SiO2-TiO2 nanoparticles formed conglomerates with visible crystalline structure and average particle size between 0.1 and 1 μm, as shown in (Figure 2a). The conglomerates exhibit a flake morphology with a flustered arrangement of the nanoparticles. A deeper analysis increasing magnification to 37,000x (Figure 2b), 70,000x (Figure 2c), and 100,000x (Figure 2d) allowed to observe individual nanoparticles with sizes ~ 10 nm grouped in 100-nm conglomerates. A further study of TEM (Figure 3) allowed to corroborate individual particle size to be of the order of 5 nm. Particle arrangement observed in TEM images suggest the presence of crystalline structure, possibly microcrystalline anatase with grain sizes of 10-30 nm as observed in previous XRD studies regarding the SiO2-TiO2 mixed oxide matrix [38-40] (the diffractograms are not shown in this paper for the sake of brevity). EDS measurements in the conglomerates showed homogeneous distribution of the elements present in the compound with atomic percentages as expected (Figure 4). The atomic composition is shown in Table 1.
Figure 2: SEM micrographs of the Cu/SiO2-TiO2 nanoparticles at 15,000 x (a), 37,000 x (b), 70,000 x (c), and 100,000 x (d). Nanoparticle conglomerate formation with sizes 0.1–1.0 μm is observed. Individual nanoparticles exhibit average particle size of 5-10 nm.
Figure 3: TEM micrographs of the Cu/SiO2-TiO2 nanoparticles with dimensions 50 nm (a, b) and 10 nm (c, d). Individual particle size was identified to be < 10 nm. The nanoparticles exhibit crystalline structures with grain sizes of 10-30 nm. Particle arrangement suggests an anatase crystalline structure.
Figure 4: EDS measurement in the conglomerates with the presence of Ti, Si, O, and Cu, elements that compose the Cu/SiO2-TiO2 nanoparticles. Carbon peaks correspond to the carbon tape in which the sample was placed.
Microcalcification accompanies several benign and malignant alterations in breast parenchyma [41,42]. Most commonly the calcifications (5 μm to 100 μm) are composed of hydroxyapatite (Ca10(PO4)6(OH)2), which forms concentric concretions and amorphous masses, [43] exhibiting grey-white, opaque, and ovoid form or fusiform shapes with irregular surfaces [44]. Less often, calcium oxalate dihydrate (Weddellite) is deposited, forming single crystals up to 1 mm long, [44,45] which are amber in color, partially transparent and form pyramidal structures with relatively planar surfaces [46]. Before treatment with Cu/SiO2-TiO2 nanoparticles and Triticum vulgare, the wound was cleaned, and intern calcifications were removed. After a few applications, internal calcifications emerged from the wound, which captured our attention. For a better understanding of the properties of the calcifications obtained, the crystals were analyzed by SEM and EDS techniques to identify morphology, texture, and composition. In (Figure 5) a sample of epithelial tissue is observed with the presence of individual calcifications with sizes of the order of 20 μm. The masses exhibit amorphous structures with no visible presence of crystal twinning. According to bibliography revised, [15,43-45] our hypothesis is that the calcifications are composed of calcium carbonate (CaCO3), calcium oxalate (Ca(CO2)), or hydroxyapatite (Ca10(PO4)6(OH)2); the aforementioned is intended to be corroborated in a further EDS study. Calcium oxalate has been shown to be associated with benign lesions of the breast or at most non-invasive lobular carcinoma in situ [47]. Calcium carbonate microcalcifications identified with breast tissues grow in calcite and aragonite crystal structures [48].
Figure 5: SEM micrographs of the calcifications observed in the wound-tissue sample. Individual calcifications with sizes of the order of 20 μm are observed surrounded by erythrocytes deposited in the surface of the epithelial tissue.
Administration of Cu/SiO2-TiO2 nanoparticles with Triticum vulgare enhanced granulation tissue formation by limiting bacterial infection as early as two months after the first application. The wound was dry and clean, with sharp edges and edema reduction. After four months of treatment, the size and depth of the chronic wound had reduced significantly, presenting an erythematous clean bed and a decrease in calcification formation. At the 6-months mark, granulation tissue was observed with optimal reepithelization and no fibrin due to revascularization and fibroblast production. After 8 months of the first application, the wound has completely healed in an atrophic scar. No inflammation was observed. The healing process is observed in (Figure 6).
Figure 6: Evolution of the chronic wound with Cu/SiO2-TiO2 + Triticum vulgare treatment at time 0 (a), after 2 months (b), after 4 months (c), after 6 months (d), and after 8 months (e). The white film observed corresponds to the zinc oxide applied as emollient.
The clinical case presented several risk factors that seriously complicated the wound healing process and represented a possible systemic recurrence: chronic untreated ulcers, hypertension, and type-2 diabetes mellitus. In healthy patients, the healing process involves coordinated interactions between diverse immunological and biological systems, which are divided into four time-dependent steps: (i) coagulation and hemostasis, (ii) inflammation, (iii) proliferation, and (iv) wound remodeling [49-55]. The four steps are essentially dependent on a proper blood irrigation to the wound for the transport of the cells (endothelial, thrombocytes, neutrophils, etc.), nutrients, and coagulation and inflammatory factors that will intervene in the process [56-59]. Diseases that lead to compromised microcirculation, such as diabetes, inhibit the delivery of oxygen and nutrients to tissue injuries, impairing wound healing [60] and causing the development of chronic wounds on account of impaired growth factor production, angiogenic response, collagen accumulation, fibrosis, and abnormal blood pressure [61]. Furthermore, due to chemotaxis and impaired leukocyte function and inadequate migration of neutrophils and macrophages to the wound, [62-64] diabetic patients develop a preponderance for infections such conditions demand for wound healing treatments that enhance reepithelization while reducing infection progression [65,66].
Titania-silica mixed oxide nanoparticles with high specific surface areas (up to 645 m2/g) have been studied regarding their catalytic properties for a broad variety of applications, [38,40,67- 69] including absorption of organic pollutants, [70] drug delivery, [71,72] and catalysis [73-75]. The incorporation of transition metals (such as platinum and copper) in the mixed matrix has been reported to enhance the antibacterial properties of the compound. [34,35] For the treatment of the chronic wounds the patient exhibited, we synthesized Cu/SiO2-TiO2 nanoparticles with catalytic and antibacterial properties. The nanoparticles were obtained by the sol-gel process through a hydrolysis catalyzer to achieve acid conditions in the gelation process, and thus, to obtain acid nanomaterials. The development of acidic properties in binary mixed metal oxides in terms of Lewis and Brønsted acidic sites has a direct impact on the catalytic properties of the nanoparticles. [38,76,77] The nanoparticles’ size observed in SEM and TEM studies (5-10 nm) makes them capable to traverse the plasmatic membrane of the bacteria through passive diffusion [78]. Once inside the bacteria, due to synthesis method used and their functionalized surface, the nanoparticles are able to catalyze the breakage of C-C and C-N bonds present in DNA and RNA nitrogenous bases hence destabilizing the structure of the molecule and inhibiting the bacterial reproduction [79]. This biocatalytic process is believed to generate molecular oxygen which enhances the proliferation of fibroblast activity, [80] collagen synthesis, [81] and inflammation, [82] hence improving the generation of granulation tissue and reepithelization, and significative decreasing the time of recovery.
The patient’s wounds treated with the Cu/SiO2-TiO2 nanoparticles exhibited total recuperation after 8 months since the first application. Through the healing process, internal solid calcifications emerged from the wound. They were removed and analyzed by SEM technique. The presence of calcium compounds in the breast is related to benign lesions, as the chronic wounds the patient exhibited. After the application of the nanoparticles, the calcification formation was reduced and eliminated as a sign of lesion recovery [41,83]. The successful outcome achieved with the treatment meant a significant improvement in the patient’s quality of life, eliminating the possibility of a systemic recurrence of breast cancer [18].
Post-breast cancer chronic wounds were successfully treated with Cu/SiO2-TiO2 nanoparticles in a patient with the menace of systemic recurrence due to risk factors such as hypertension and type-2 diabetes mellitus. Good outcomes were observed in terms of tissue regeneration and wound healing. The therapy with the nanoparticles embedded in a polymeric gel hindered infection formation allowing wound reepithelization and healing with a time of recuperation significantly reduced (8 months in a 30-years-old chronic wound). Furthermore, calcifications present in the wound were removed and the recurrent formation was eliminated after the treatment. No adverse effects were observed in the patient. Scientific evidence supporting the efficacy and safety of the Cu/ SiO2-TiO2 therapy is hereby shown. The nanoparticles can be used as a primary apposite to stimulate the autolytic debridement of injures, due to their physical-pharmaceutical properties: excellent local absorption because of its nanoparticulate composition and bactericide action that helps control local infections. Yet, future studies must be carried out to further confirm the efficacy of the nanoparticles.
a. Microcalcification formation elimination is related directly with the catalytic effect of the nanoparticles or with the wound healing process?
b. Do we need to assess the effectiveness the nanobiocatalyst
in the real world and not simply rely on clinical trial data?
c. What can be done to improve wound healing rates?
d. How can the efficiency of health-care delivery be
improved?
All authors contributed to the study conception and design. The design and synthesis of the nanoparticles were executed by TL, PR and FJPG. The characterization techniques were made by EGL. The patient’s treatment was carried out by VSB. The first draft of the manuscript was written by FJPG. All authors commented on previous versions of the manuscript and critically revised it. FJPG was responsible for compiling the final draft. All authors read and approved the final draft.
This study was funded by the Autonomous Metropolitan University – Xochimilco and NANOTUTT S.A. de C.V.
Conflict of Interest: The authors declare that they have no conflict of interest.
Ethics Approval: This study was performed in line with the principles of the Declaration of Helsinki. Necessary ethical clearance was obtained from the ethical committee office code number JUDI- 01/07 CDMX under the protocol: [“Security and efficacy of the local application of Pt/SiO2-TiO2 nanomaterials in patients with diabetic foot ulcers” Education and Investigation Direction], and the study was conducted in accordance with the ethical Good Clinical Practice.
Consent to participate. Informed consent was compulsory for contribution in the study. The staff notified the contributor with the objectives, dates, drugs, diet, possible risks, and general activities through the clinical study.
Consent to Publish: No personal data of the patients was mentioned in the study.
1. Tessy López: https://orcid.org/0000-0002-6048-0419
2. Francisco J. Padilla-Godínez: https://orcid.org/0000-
0002-9253-2463
Bio chemistry
University of Texas Medical Branch, USADepartment of Criminal Justice
Liberty University, USADepartment of Psychiatry
University of Kentucky, USADepartment of Medicine
Gally International Biomedical Research & Consulting LLC, USADepartment of Urbanisation and Agricultural
Montreal university, USAOral & Maxillofacial Pathology
New York University, USAGastroenterology and Hepatology
University of Alabama, UKDepartment of Medicine
Universities of Bradford, UKOncology
Circulogene Theranostics, EnglandRadiation Chemistry
National University of Mexico, USAAnalytical Chemistry
Wentworth Institute of Technology, USAMinimally Invasive Surgery
Mercer University school of Medicine, USAPediatric Dentistry
University of Athens , GreeceThe annual scholar awards from Lupine Publishers honor a selected number Read More...