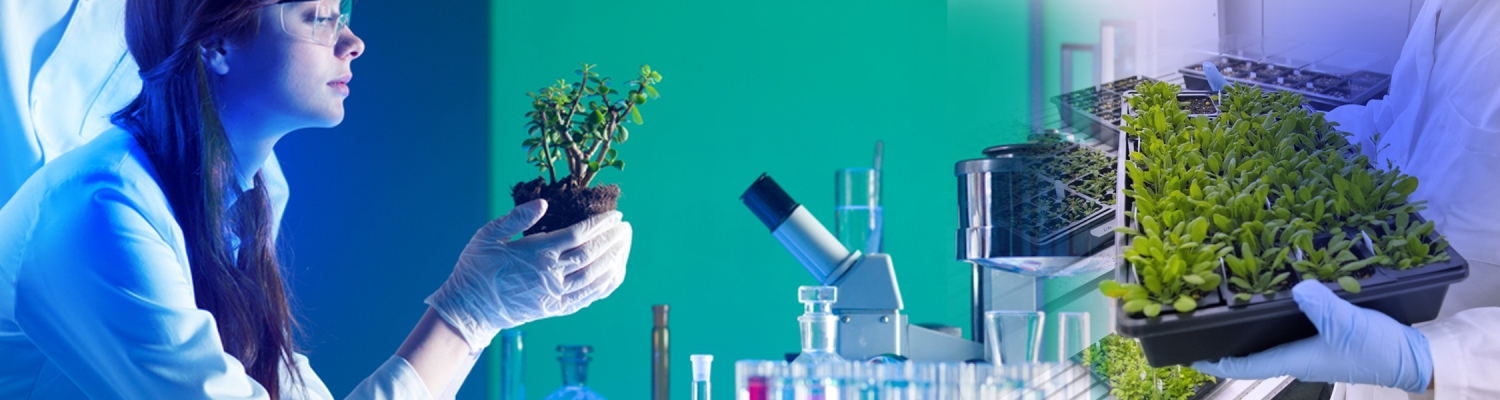
ISSN: 2641-6794
Shiguo Wu*, Hao Zhou, Jun Wu and Chengxian Liu
Changzhou Institute of Engineering Technology, Changzhou 213164, PR China
Received: November 18, 2019; Published: November 25, 2019
Corresponding author: Shiguo Wu, Changzhou Institute of Engineering Technology, Changzhou 213164, PR China
DOI: 10.32474/OAJESS.2019.04.000181
Low temperature denitration technology is a hotspot in recent years. Manganese-based catalysts are demonstrated to be most promising catalysts at low temperature. Many researchers have improved manganese-based catalysts from different aspects to meet the requirements of low-temperature catalysts. This paper attempts to review the progress of manganese-based catalysts from catalyst morphology, catalyst carriers, composite manganese-based catalysts and catalyst preparation methods in recent years, and the existing problems are also analyzed in the review.
Keywords: Low-temperature denitration; Manganese-based catalysts; NH3-SCR
Atmospheric contaminant treatment becomes an important research topic in recent years with the strict requirements of environmental protection. Nitrogen oxides (NOx), which emitted from stationary sources (e.g., coal-fired power plants) and mobile sources (e.g., vehicle exhausts), is one of the major air pollutants. It can cause not only photochemical smog and acid rain, but also ozone depletion and greenhouse effect [1]. Many methods are used in applied and fundamental studies about denitration catalyst. Among them, The Selective Catalytic Reduction of NOx by NH3 (NH3 -SCR) is to date the best developed and most widespread method for NOx removal from stationary sources, and the general NH3-SCR reaction is: 4NO + 4 NH3 + O2= 4N2+6H2O [2]. Commercial technology, which employing V2O5-WO3 (or MoO3)/TiO2 as catalyst [3], exhibits excellent catalytic performance in the temperature range of 300-400 ℃. However, there are still some disadvantages as follows [4-7]: (1) V2O5 is easy to sublimate in the using process, which causing biological toxicity when it enters into the environment; (2) SO2 durability is unsatisfactory. The low temperature denitration technology (<200 ℃) can place the SCR device in the low temperature and low dust section at the end of the flue gas treatment system, which can reduce the poisoning and mechanical wear of the catalyst. It is a meaningful work to develop low-temperature NH3-SCR catalysts, therefore, many efforts have been made to develop novel non-vanadium-based metal oxide denitration catalysts with merits of high low-temperature efficiency and environment friendly.
Many investigations have been carried out on low-temperature NH3-SCR reaction. In comparison, manganese species have been demonstrated to be higher NOx conversion at low temperatures. Pena et al. [8] studied the removal of NO by transition metal oxide/TiO2 under low temperature conditions and found that the denitration efficiency of different metal oxides is Mn>Cu>Cr>Co>Fe>V>Ni. It is believed that the transfer of electrons between different valence manganese species is very rapid, and it is conducive to improve NH3-SCR low-temperature activity. Huang et al. [9] prepared pure MnOx (MnO, Mn3O4, Mn2O3, MnO2) catalysts in NH3-SCR reaction, the results showed that MnO2 is more suitable than other manganese oxides. Kapteijn et al. [10] studied the denitration performance of MnOx with different valence states, and the conclusion is similar to Huang & Luo et al. [11] loaded MnO2 with different crystal on TiO2-palygorskite (TiO2-Pal) and found that γ-MnO2 gave higher activity than δ- MnO2 and γ- MnO2. MnO2 particles had a better dispersion in the carrier when mass ratio of Pal to TiO2 was 2:1.
Ordinary manganese-based catalysts cannot meet the requirements of low temperature catalysis. In recent years, many researchers have improved manganese-based catalysts from different aspects to meet the requirements of low-temperature catalysts. This paper attempts to summarize the progress of manganese-based catalysts from catalyst morphology, catalyst carriers, composite manganese-based catalysts and changing catalyst preparation methods in recent years.
Change catalyst morphology Tian et al. [12] prepared different morphologies MnO2 using different methods. The results indicated that nanorods MnO2 contributed the better denitration effect, and it was due to the large amount of strong acid sites, low crystallinity and more lattice oxygen. Hu et al. [13] prepared Mo(x)-MnOx rectangular nanorod catalyst by co-precipitation method. The corresponding catalytic performance and anti-H2O performance increased significantly. Moreover, nanorod-formed catalysts have higher concentrations of Mn4+, richer surface adsorption of oxygen (promoting NO oxidation to NO2 and "fast-SCR" processes) and active sites. Also, the Mo(x)-MnOx nanorod catalyst has stronger acidity to adsorb ammonia species, which is also beneficial for increasing the catalytic activity of the catalyst. Li et al. [14] prepared MnFeOx nanorods used a hydrothermal method, and the results indicated that the MnFe0.1Ox nanorods exhibited the highest NO conversion rate in NH3-SCR reaction. It was due to MnFe0.1Ox nanorod catalysts obtained higher oxidation activity of NO to NO2. Li et al [15] prepared a three-dimensional flower-like NiMnFeOx mixed oxide catalyst and found that the structure of the three-dimensional flower-like NiMnFeOx mixed oxide can improve the NH3-SCR performance significantly. Li et al. [16] prepared a CeO2-MnOx catalyst with core-shell structure by chemical precipitation method, and the denitration rate reached over 90% at 110~220 °C.
Supported manganese-based catalysts YAO et al. [17] investigated the catalytic performance of manganese species on different supports and established a related reaction model. The results show that γ-Al2O3 can provide the highest dispersion, most acidic sites and excellent NOx absorption capacity. Huang et al. [18] loaded Mn and Fe oxides onto mesoporous SiO2 with Mn/Fe mass ratio of 1 and calcination temperature at 400 ℃, and 99.1% NO conversion rate was achieved at 160 ℃, and H2O has little effect on the catalytic activity. Guo et al. [19] prepared Ce-MnOx/Al2O3 catalysts using impregnation method. The denitration performance of the catalysts in the range of 70~300 ℃ was investigated. The results showed that Ce addition can improve the dispersion of MnOx on the carrier surface. 4% Ce-MnOx/ Al2O3 showed good denitrification efficiency. The effect of calcination temperature on the catalytic performance was also investigated, and it found that the catalyst calcined at 550 ℃ contributed the best catalytic performance.
Composite manganese-based catalysts with the development of low temperature SCR catalyst, complex transition metal oxidation catalyst has been studied. Among these catalysts, the composite Mn-based catalysts were of great importance because of its wider active temperature range and higher denitration efficiency. Li et al. [14] prepared MnFeOx bimetallic oxides catalysts resulting in excellent SCR catalytic activity. The BET surface area of MnFeOx catalysts was larger than that of pure MnO2. The authors attributed the results to a redox reaction may occur between Mn and Fe ions resulting in electronic transfer. The electronic transfer played a key role in the oxidation of NO to NO2 during the NH3-SCR reaction of removing NOx. Zuo et al. [20] prepared Mn-Zr catalyst and found that MnOx was better dispersed when Mn/Zr molar ratio was 0.5, and the catalytic activity was strengthened due to the synergistic effect between Mnn+and Zr4+. Liu et al. [21] added WO3 to the Mn-Zr catalyst. The highly dispersed WO3 not only increased the acid sites on the catalyst surface, but also inhibited the adsorption of inert nitrates onto the catalyst surface. Wan et al. [22] synthesized NiMnOx catalysts with different molar ratios by co-precipitation. The catalyst showed highest denitration activity when the molar ratio of Ni/Mn was 0.4. It was illustrated that the catalytic performance was promoted due to the synergistic effect between Ni and Mn.
Guo et al. [23] studied the Sb modified MnTiOx catalyst and found that the average pore size of the catalyst increased due to the presence of Sb, resulting in a corresponding increase in the surface area of the catalyst. Wu et al. [28] found that adding a transition metal to the Mn/TiO2 catalyst can improve the dispersibility of the catalyst. Wu et al. [24] prepared a series of Ce-modified MnTi catalysts and evaluated the activity of catalysts with different Ce contents. The results indicated that the addition of Ce can improve the activity of the catalyst. When the Ce content was 0.07%, the catalyst contributed the highest catalytic activity. Through the analysis of TPR and XPS, the authors found that Ce modification increased the chemical adsorption ability of oxygen and acidic sites on the surface of the catalyst, which were beneficial to the catalytic reaction. Thirupath et al. [25] doped Ni to MnOx/TiO2 catalyst. The results showed that Ni doping improved the denitration efficiency. The presence of Ni enhanced the stability of active Mn4+ in the catalyst, inhibits the formation of Mn3+, and improved the H2O and SO2 resistance. Shen et al. [26] doped Fe in Mn-Ce/TiO2, and the catalyst showed good activity when the molar ratio of Fe/Ti was 0.1. The addition of Fe also improved the resistance ability of H2O and SO2 for the catalyst, and resistance ability was strengthened with the increase of Fe content until Fe content was 0.15%. Huang et al. [27] studied in detail the modification effect of Fe on Mn/mesoporous silica catalysts. It was found that the addition of Fe greatly improved the denitration performance of the catalyst, especially when the molar ratio of Fe to Mn was 1:1. The conversion of NO increased by about 40% at 100 °C; the characterization results show that the addition of Fe mainly improves the thermal stability and dispersion of Mn. Qiu et al. [29] studied the denitration performance of Co-Ce-Mn/TiO2 catalysts formed by the addition of Co and Ce. It was found that the addition of Co and Ce increased the redox and the acidity of the catalyst compared with the Mn/TiO2 catalyst. The addition of Co will produce a large amount of B acid sites, which is beneficial to the adsorption of NH3, and the addition of Ce is beneficial to the formation of NH4+. Qi et al. [30] investigated the effects of the addition of different elements (Fe, Cu, Zr and Pr) on the activity of MnOx-CeO2 catalyst and N2 selectivity. The results showed that the addition of Fe and Zr could significantly increase the denitration activity of the catalyst in the range of 100-180 °C, but the N2 selectivity did not change. The addition of Pr was opposite, which could increase the selectivity of N2 and reduce denitration activity. Cu has no significant effect on denitration activity and N2 selectivity. These changes are determined by the reduction performance and chemisorption capacity of the catalyst.
Changing catalyst preparation methods Tang et al. [31] synthesized MnO2 catalysts with different methods. It was found that MnO2 prepared by solid phase method and rheological method exhibited excellent low temperature activity, and the conversion rate of NOx was close to 100% at 100 ℃. At the same time, the analysis showed that the amorphous state of the catalyst was the key factor for the low temperature catalytic activity. Jiang et al. [32] investigated the effects of MnO2/TiO2 preparation methods (sol-gel method, impregnation method, co-precipitation method) on its denitration performance, and found that the catalyst prepared by sol-gel method showed the best performance, active and anti-SO2 properties. In addition, the crystal form, specific surface area, and calcination temperature of MnOx are also important factors affecting the low temperature denitration performance [33]. Zhang et al. [34] prepared MnOx/TiO2 catalysts using conventional impregnation method, ultrasonic impregnation method and sol-gel method, and employed them in SCR reactions. The results revealed that the catalyst obtained by ultrasonic impregnation gave the best catalytic activity at low temperature.
The conversion rate of NO was more than 90% at 120 ℃. The other two catalysts gave comparable denitration efficiency almost at 150 ℃. The results illustrated that the sample using ultrasonic impregnation method exhibited higher dispersibility for active species, and more Lewis acid sites, which improved the catalytic performance. France et al. [35] synthesized Ce-Fe/MnOx catalyst by citric acid method. The denitration rate of Ce12.5-Fe/MnOx was over 97% at 105~135 °C, and denitration rate decreased only 4% after passing into SO2. Shi et al. [33] synthesized TiO2 with multi-stage pore structure (macroporous-mesoporous) and studied the effect of pore structure on the denitration performance of the catalyst. Compared with the non-multi-stage pore Mn/TiO2 catalyst, multi-stage pore Mn/ TiO2 exhibits high low temperature denitration activity and strong sulfur resistance. The authors attributed it to the larger specific surface area and the dispersion degree of the active component Mn. In our previous work, FeMnTiOx mixed oxides catalyst, which was synthesized with CTAB-assisted and calcined at 500 ℃, showed excellent low-temperature performance in NH3-SCR reaction [36].
In summary, relevant research on low temperature denitration catalysts has made some progress. Compared with other types of low-temperature denitration catalysts, manganese-based catalysts have high denitration efficiency and low cost, which can basically meet the denitration needs of coal-fired power plants. However, at present, there are still many problems in low temperature denitration catalysts. For example, there are a certain amount of SO2 and water vapor in various types of flue gas. Manganese-based catalysts exhibit poor denitration activity when H2O and SO2 coexist. Furthermore, the related mechanism of low temperature denitration catalyst needs to be gradually elucidated, and the working temperature window of the catalyst needs to be widened.
Bio chemistry
University of Texas Medical Branch, USADepartment of Criminal Justice
Liberty University, USADepartment of Psychiatry
University of Kentucky, USADepartment of Medicine
Gally International Biomedical Research & Consulting LLC, USADepartment of Urbanisation and Agricultural
Montreal university, USAOral & Maxillofacial Pathology
New York University, USAGastroenterology and Hepatology
University of Alabama, UKDepartment of Medicine
Universities of Bradford, UKOncology
Circulogene Theranostics, EnglandRadiation Chemistry
National University of Mexico, USAAnalytical Chemistry
Wentworth Institute of Technology, USAMinimally Invasive Surgery
Mercer University school of Medicine, USAPediatric Dentistry
University of Athens , GreeceThe annual scholar awards from Lupine Publishers honor a selected number Read More...