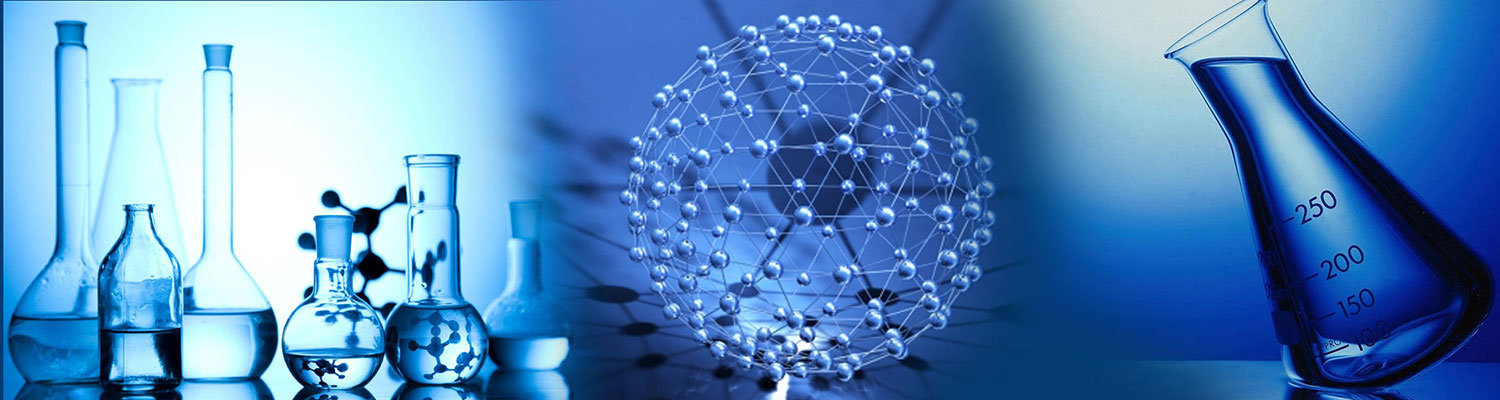
ISSN: 2637-4609
Ibtisam Kamal*
Received: January 25, 2019; Published: August 06, 2019
*Corresponding author: Ibtisam Kamal, Chemical Engineering Department, Faculty of Engineering, Soran University, Kurdistan Region, Iraq
DOI: 10.32474/AOICS.2019.04.000184
The objective of this article is to thoroughly review the published literature, and to amalgamate and summarize the current state and disparate knowledge regarding classification, preparation methods, functionality and industrial applications of polymer films and coatings and in so doing, not only was thorough review of current knowledge, but current gaps in the knowledge base were also identified and highlited. New approaches in developing and utilization of edible fims and products having potential in food preservation are covered. Researches into the manufacture of edible fims using a wide range of natural polymers: mono and mixed, linear and crosslinked including hydrophilic and hydrophobically modified polyelectrolytes using different technologies have been included.
Keywords: Edible films and coatings; Food preservation; Hydrophilic polyelectrolytes; Hydrophobically modified polyelectrolytes
Food packaging is meant for protecting food from external influences as well as to provide mechanical supporting for nonsolid food. The principal objectives of food packaging is to retard deterioration, extend of shelf-life, and maintenance of quality and safety of the packaged food.
In general, packaging options have expanded from the traditional materials of glass, metal and paper and synthetic polymers engineered to meet particular requirements. Petrochemical-based plastics have advantages in their availability in large quantities at a low cost and good functionality such as tensile and tear strength. However, increased use of synthetic packaging films based on petrochemicals has led to serious ecological problems due to their total non-biodegradability. Thus, consumer demand has shifted to safe and eco-friendly biodegradable materials, especially from renewable agriculture by-products and food processing industry wastes. Motivated to this, new and novel food-grade packaging materials and technologies have been and continue to be developed based on bio-based polymers.
It is well established that polymers have low unit weight and good shear strength characteristics and thus hold promise for flowable fill applications. Use of natural biopolymers for diversified applications in life sciences has several advantages, such as availability from replenishable agricultural or marine food resources, biocompatibility, biodegradability, therefore leading to ecological safety and the possibility of preparing a variety of chemically or enzymatically modified derivatives for specific end uses. The development and characterization of edible films and coatings have increasly attracted the attention of biochemists, biotechnologists, and physicists, among others, mainly to the large variety of applications served by bio-based polymers [1,2].
Some polymers derived from natural sources have been engineered for certain products and are already in the market place. Some of these polymers are edible and have played and continue to play an instrumental role in food throughout history and in the food, pharmaceutical and other industries. Many of these polymers are used alone or in combination with synthetic polymers to create a class of active packaging commonly referred to as edible films or coatings [3].
Edible films and coatings afford numerous advantages over conventional non-edible polymeric packaging. They can reduce the complexity of the food package and, even if they are not consumed with the packaged product, can contribute to the reduction of environmental pollution by virtue of their biodegradable nature.
Edible coatings can be prepared using two-process approaches [4]. The dry-process approache, such as thermoplastic extrusion, based on the thermoplastic properties of polymers when plasticized and heated above their glass-transition temperature in low watercontent conditions. The disadvantage of extruded films is that they cannot be used to cover irregular surfaces. The second approach is the wet-process approach, which is based on a film-forming dispersion in which polymers are first dispersed into a liquid phase, and then dried at the end of the application. The wet process permits the application of coating in liquid form directly onto food products by dipping, brushing or spraying [5].
It is well known most of the foods are susceptible to mechanical damage, physiological deterioration, water loss and decay during storage. Reduction in turgidity as a result of water loss causes shrivelling and faster depletion of nutrients and organoleptic properties, and is a major cause of deterioration. Cold storage of some foods is common, but other foods such as fruits are usually stored in markets at room temperature between harvest and consumption. For this reason, product losses are very high. With the use of edible coatings and cold storage, spoilage could be minimised. Edible films have been applied to fruits, vegetables [6], meat, poultry, grains, and confectionery products, heterogeneous foods, any of which can be fresh frozen, cured or processed.
The most important functionalities of an edible film or coating include control of mass transfers, mechanical protection, and sensory appeal. Control of mass transfers involves preventing foods from desiccation, regulating microenvironments of gases around foods, and controlling migration of ingredients and additives in the food systems. Edible coatings on freash foods can provide an alternative to modified atmosphere storage by reducing quality changes and quantity losses through modification and control of internal atmosphere of indivitual foods. Modification of internal atmospher by the use of edible coatings can increase disorders associated with high carbon dioxide or low oxygen concentration, even though ingress of oxygen may reduce food quality owing to oxidation of the aroma components in the food. Also edible film with greater water vapour permeability is desirable for freash products, although extremely high water vapor permeability is also not desirable as it can result in excessive moisture loss of fruit during storage. Adequate mechanical strength of an edible film is necessary to protect the integrity of packaging throughout distribution. The sensory properties of an edible coating or film are a key factor for acceptance of final products.
One of the most important factors in the preparation of edibe films regards the choice of ingredients. Edible coatings and films based on polysaccharides a class of natural macromolecules, have the tendency to be extremely bioactive, and are generally derived from agricultural feedstock or crustacean shell wastes. Polysaccharides have been mainly used for food covering due to their excellent selective permeability to oxygen and carbon dioxide. These low-cost films are mostly prepared with cellulose and its derivatives such as ethers and esters, starch, pectins, and gums which are of applications in food preservation. Edible coatings based on cellulose have been extensively applied to delay loss of quality in fresh fruit products such as tomatoes, cherries, fresh beans, strawberries, mangoes and bananas. Chitosan is another polysaccharide widely used in the post-harvest decay control of fresh fruits and vegetables. Chitosan is derived from chtin which is next to cellulose [7].
Polysaccharides capable of forming gels in water are common throughout the plant kingdom. Some of them, such as the pectins in higher plants, carrageenans and agarose in algae, algal and bacterial alginates and xanthan, have been investigated in great detail. Mucilages are generally hetero-polysaccharides obtained from plant stems such as the waste product cactus stems. Cactus mucilage may find applications in food, cosmetics, pharmaceutical and other industries [8]. The complex polysaccharide is part of dietary fibre and has the capacity to absorb large amounts of water, dissolving and dispersing itself and forming viscous or gelatinous colloids. An important point in the choice of the cactus mucilage as a coating is its low cost.
Polysaccharides edible coatings showed different functionalities. Pectin-based edible coatings containing green tea leaf extract powder applied on cooked pork patties irradiated by Gamma ray showed decreased lipid oxidation and increased radical scavenging properties [9]. Edible coatings made from hydroxypropyl methylcellulose containing surfactant mixtures in aqueous and hydroalcoholic media were developed. The water vapour permeability of the films were increased with hgh viscosity, low surface tension, great lipid particle size,and high flocculation rate of the film-forming dispersions [10]. Composite edible films using polysaccharide mixtures of ager- starch- arabinoxylan, shows that agar can to be an advantageous component that contributes to the improvement of the mechanical properties of starch films. Besides, arabinoxylan could be considered as an additive for ager based films to increase moisture barrier efficiency [11]. Hydroxypropylmethylcellulose edible coatings containing an ethanolic extract of propolis were developed and applied to grapes fruit. Propolis incorporation was found to increase the color luminosity of the grapes and to enrich the nutritional characteristics of the coated product [12]. Intensive work has been carried out by on different kinds of polysaccharides including cellulose derivatives and other composite coatings. Promising results were obtained by their applying on fruits and vegatables within a thermomechanical pre-treatment step (DIC: Instant Controled Pressure Drop Process) [13]. Rich information in this concern is available in the literature [14-16].
On another hand, proteins, such as casein, whey proteins and corn zein, have also been used as in edible coatings a moisture barrier since these proteins are abundant, cheap and readily available. Protein-based films have been prepared with both vegetal and animal proteins, including corn zein, soy protein, wheat proteins (gluten, gliadin), peanut protein, gelatine, casein, and milk whey proteins [17]. Food protein can supplement the nutritional value of the food , they may act as natural vehicles, evolved to deliver essential micronutrients (e.g. calcium and phosphate) and building blocks (e.g. amino acids), as well as immune system components (e.g. immunoglobulins, and lactoferrin [18]. Besides, food proteins can be used in coating formulations to develop easily degradable packaging, non-aggressive to the environment, capable to be applied to different kind of foods including vegetables, fruits, poulitry and fish products [19]. Protein-based edible films are attractive because they have impressive gas barrier properties compared with those prepared from lipids and polysaccharides. However, the poor water vapor resistance of protein films and their lower mechanical strength in comparison with synthetic polymers limit their application in food packaging. Hence, improvement of edible protein film properties has been investigated to seek suitable applications. The methods used to improve the mechanical strength and moisture barrier properties of protein based edible coatings are reviewed else where [20].
Inspite of the potential of multi-funtional properties of polysaccharides and proteins, the hydrophilic nature of these biopolymers limits their ability to provide the desired edible film functions. Several approaches to improve water barrier and mechanical properties of these films include incorporation of hydrophobic compounds, optimisation of interaction between polymers and formation of cross-links. Lipid compounds include neutral lipids of glycerides which are esters of glycerol and fatty acids, and waxes which are esters of long-chain monohydric alcohols and fatty acids, are commonly utilized in edible coatings. They are added to food coatings to impart hydrophobicity [21]. Figure 1 shows the various work concepts in manufacturing, characterization and properties of edible coatings.
Edible films and coatings, can be divided into proteins, polysaccharides, lipids and composites. They are defined as thin layers formed on a food surface as a coating, or placed (pre-formed) between food components. Their purpose is to extend the shelf-life of the food product and provide a barrier against hazards. They can retard moisture migration and the loss of volatile compounds, reduce the respiration rate, and delay changes in textural properties. Also, they are excellent barriers to fats and oils, and have a high selective gas permeability ratio CO2/O2 as compared to conventional synthetic films [14,22]. They can also act as carriers of food additives such as antioxidants [23] and/or antimicrobial agents [24] and can improve mechanical integrity or handling characteristics of the food. Stand-alone edible films with good mechanical properties could replace synthetic packaging films for specific applications.
Over the last ten decades, extensive researches have been undertaken on the use of aqueous-based film coatings. Potential sources, performance and applications of these coatings have been reviewed throughout huge number of publications, but water-insoluble edible coatings have gotten less attention. Ethyl cellulose and other polymer composites of polysaccarides with improved water barrier properties have been developed throughout formulating of biopolymer composites based on the hydrophilic polysaccharides and reducing the hydrophilic nature of the polysaccharides by different methods including incorporation of hydrophobic compounds, interaction between the biopolymers and formation of cross-links.
Pectin, chitosan and sodium alginate are selected as the examples of hydrophilic polyelectrolytes. The chemical structures of chitosan, pectin and algenic acid are shown in Figure 2.
Pectin
Pectin is one of the proportionally largest materials in citrus by-products. It is a natural, non-toxic and anionic polysaccharide extracted from cell walls of most plants and used as gelling and thickening agents in food technology and as a drug carrier in pharmaceutical research [18,25]. It is predominantly a linear polymer of mainly α-(1→4)-linked d-polygalacturonic acid residues. They are structurally complex and heterogeneous polyelectrolytes consisting of linear regions of (1→4)-α-d-galacturonosyl units and their methyl esters, interrupted in places by (1→2) α-lrhamnopyranosyl units. The degree of methoxylation (DM) is used to classify the pectins as high methoxyl pectins (DM>50) and low methoxyl pectins (DM<50). The application and evaluation of pectin-based coating on the kinetics of quality change in stored fruits using a pre-established coating process was highlited in literature [26].
Chitosan
Some polymers are inherently antimicrobial and have been used in films and coatings such as the cationic polymer chitosan since charged amines interact with negative charges on the cell membrane, causing leakage of intracellular constituents [27]. Chitosan has been used as a coating and appears to protect fresh vegetables and fruits from fungal degradation. Although the antimicrobial effect is attributed to antifungal properties of chitosan against pathogenic and spoilage micro-organisms, including fungi, and both Gram-positive and Gram-negative bacteria [28], it may be that the chitosan acts as a barrier between the nutrients contained in the produce and microorganisms [29] as well as as a carrier in drug delivery [30]. In addition, chitosan-based antimicrobial films have been used to carry bioactive molecules [31].
Chitosan has another one important property that it can undergo chemical modification very easily. This property is attributed to the presence of free amino groups, which increases the reactivity of the polymer. Chitosan can therefore be readily modified by reactions at the amino groups. The use of the optimal concentration of glycerol, in chitosan-based films, improved the mechanical properties of the films and allowed to obtain the best water vapour permeability value. The addition of the optimum content of tannic acid, to the chitosan matrix led to form a more rigid structure because it acted as a crosslinking agent [32]. Chitosan – Methyl cellulose combined biodegradable films were found to be of good water vapor barrier properties and have the possibility to tailor mechanical and solubility properties, within a range, based on polymer ratio composition. This property has helped researchers to improve the chemical and mechanical properties of chitosan to many possible applications in food, pharmaceutical and cosmetic industry [33]. A water-soluble chitosan derivative, O-fumaryl-chitosan was prepared by using the selective partial acylation of chitosan and fumaric acid in the presence of H2SO4, the resulted polymer had good solubility in a wide pH range, which was related to the degree of substitution. It showed higher microbial activity, giving it the potential of becoming alternatives for food preservation instead of some harmful bactericides [34]. Further more, chitosan and poly (DL-lactide-co-glycolide) were used to fabricate biodegradable porous scaffolds for applications in tissue engineering by thermally induced phase separation technique [35]. Edible film made of cassava starch, glycerol and chitosan showed that optimal transparent white films with no bubbles were obtained on the temperature variable 80 °C and 3 hours of drying time [36]. Chitosan from meti shell showed potential use as an edible film polymer with desirable properties [37].
Alginate
Alginate was originally thought to consist of a uniform polymer of mannuronic acid. However later studies showed that alginic acid is a linear copolymer of 1,4-linked β--mannuronic acid (M) and α--guluronic acid (G). Algenic acid it is a high-molecularmass polysaccharide extracted from kelp. It has been shown that the G and M units are joined together in blocks and as such, three types of blocks may be found: homopolymeric G blocks (GG), homopolymeric M blocks (MM) and heteropolymeric sequentially alternating blocks (MG).
Alginate based coatings have potential applications in edible coating field, among which, they are used as carriers for antioxidants for shelf-life extention of fish [23] and as antibrowning agents for fresh-cut fruits [16].
Hydrophobically modified polyelectrolytes
When aqueous systems of different biopolymers are mixed together, the behavior of the individual polymer is usually affected and a synergism between the polymers emerges. The interaction between unlike chains can be either more favorable or less favorable than interactions between like chains of each type. Mixed biopolymers are used in a wide range of applications, because of their ability to interact synergistically and provide material with controlled properties [1].
Water soluble polymers are well-known for their thickening properties in aqueous solutions. High molecular weight polymers or polyelectrolytes are used as viscosifiers, but these polymers could exhibit a loss of their viscosifying properties when they are submitted to strong mechanical deformations, increasing ionic strengths or high temperatures. To overcome these drawbacks, incorporation of a few hydrophobic groups in a hydrophilic macromolecular chain can lead to profound changes in the physicochemical behavior of the parent macromolecule, because of intraand/ or intermolecular associations of the hydrophobic groups [38,39]. The result is a water soluble system which self-aggregates in water, resulting in thickening effects. A simple example of hydrophobically modified polyelectrolytes is one that consist of a long hydrophilic chain (a polysaccharide such as starch) to which small amounts of hydrophobic substituents (a protein) are attached or incorporated [40]. The attachment of hydrophobic side chains onto a polyelectrolyte backbone can increase emulsion stability and heat and freeze stability. Figure 3 shows the chemical structures of hydrophobic modified chitosan and hydroxyethyl cellulose.
The hydrophobic modified biopolymers are able to associate and form highly viscous solutions or strong hydrogels in pure water. The hydrophilicity–lipophilicity relationship of such polymers depends on the degree of substitution, i.e. the content of non-polar substituents attached to the original polar macromolecule [41].
Crosslinking reactions are often used to form polymeric networks demonstrating high gel strength. When polymerization proceeds in a three-dimensional manner, some period after it has progressed to a certain threshold, gelation occurs. This well-defined transition during polymerization is known as the gel point. At this point the reaction mixture changes from a viscous liquid to an elastic gel. The amphiphilic nature imparted upon polysaccharides after hydrophobic modification give them a wide and interesting applications spectrum, as rheology modifier, emulsion stabilizer, surface modifier and as drug delivery vehicles as well as in the field of edible coatings.
Pectin complexes
Pectins can gel in various ways depending on the type and structure of the pectin molecule. Gelling can be induced by acid, by cross-linking with calcium ion, through the oxidization of the feruloylester substituents on sugar beet pectin, or by synergistic reaction with alginate. Acid-inducing and calcium cross-linking pectin gels have been used most often for the development of pectin-based drug delivery systems [42]. Pectin with a low degree of methylation forms gels in the presence of calcium ions whereas pectin with a high degree of methylation forms gels in acidic media with the addition of different sugars, e.g., sucrose or glucose [43].
Calcium salts or multivalent cations can crosslink the galacturonic acids of pectin main polymer chains; as a result calcium pectinate is obtained by the formation of an ionic bond between the carboxylic acid groups of the pectin molecules and the calcium ions [44]. Cross-linking by calcium ions have been investigated as methods for reducing the inherent solubility. Calcium pectinate has been studied and has been indexed as a hydrophilic coating agent which is insoluble when prepared according to the interfacial complexation process. Calicium pectinate solution can be ued as edible coat to food. The coating material is applied using immersion coating process, the food cileces are first immersed in pectin solution; filtrated and then immersed in cold solution of calcium chloride dihydrate solved in water used as the external calcium cross-linker. Finally, they were dried.
It was addressed that the lower the degree of methylation of the pectin the more sensitive the pectin is to calcium as a gelling agent [45,46]. The geling properties of low-Methoxyl Pectinate received significant attention in the development of edible coatings having potential use in osmotic dehydration [47,48].
Chitosan–alginate complexes
Chitosan–alginate complexes are oppositely charged polyelectrolytes. Alginate has the property of shrinking in low pH and getting dissolved in higher pH, whereas chitosan dissolves in low pH and is insoluble in higher pH ranges. In view of these concept of alginate–chitosan polyelectrolyte complexes gained acceptance, upon mixing, the carboxyl residues of alginate and the amino groups of chitosan ionically interacts to form the polyelectrolyte complex. The easy solubility of chitosan in low pH is prevented by the alginate network since alginate is insoluble in low pH conditions. The possible dissolution of alginate at higher pH is prevented by the chitosan which is stable at higher pH ranges. Multilayer films or coatings made by successive adsorption of the nanometric polyelectrolytes of (chitosan/ alginate) on a solid carrier showed to have enhanced mass transfer and mechanical properties that may find promising applications in biomedical and food fields [49,50].
Chitosan–pectin complexes
Pectin can form an interpolymer complex with chitosan. Mixtures of pectin and chitosan have also been used as film. Polyelectrolyte complex film between pectin as an anionic polyelectrolyte and chitosan as a cationic species was prepared by blending two polymer solutions [46,51,52]. Chitosan is ionised (pKa=6.0) at pH 1.1 as, to some extent, is pectin (pKa≈3.0). Both materials are soluble at this pH and an interaction would be expected between the carboxyl groups of the pectin and the amino groups of the chitosan. In basic medium, the protection offered by the coat should be mainly due to the pectin as chitosan is insoluble at this pH.
Combinations of pectin and chitosan form a polyelectrolyte complex at pH values in the range of 3–6. In addition to the formation of a polyelectrolyte complex, pectin and chitosan also interact by hydrogen bonding at low pH values (pH<2). At these pH values, pectin will be unionized and the importance of electrostatic interactions is suppressed, and an interaction between pectin and chitosan will probably take place via hydrogen bonding [53]. Chitosan-Arabic gum complex was used to prepare a biofungicide coating for banana [24].
Ethyl cellulose-pectin complexes
The major problem encountered with pectins, is their solubility and swelling properties in aqueous media. To overcome the problem of dissolution of pectin, many approaches have been evaluated, among these approaches, the combination of pectin with water insoluble polymers. The arised film coating materials from this combination showed promising results [24,54]. As a consequence the incorporation of appropriate amounts of pectins in the commercial insoluble ethyl cellulose aqueous dispersions could be an interesting alternative and could provide protection coatings [55].
Calcium alginate
The reactivity of sodium alginate with calcium and the subsequent gel formation capacity is a direct function of the average chain length of the G blocks. Hence, alginates containing the highest GG fractions possess the strongest ability to form gels. This initially arises from the ability of the divalent calcium cation, Ca2+, to fit into the guluronate structures like eggs in an eggbox. Consequently, this binds the alginate chains together by forming junction zones, and sequentially leading to gelling of the solution mixture and pellet formation. The application of these physicochemical characteristics in the formulation of conventional calcium–alginate encouraged to design of formulated binary calcium–alginate–pectinate systems [56]. Moreover, calcium alginate films were found to reduce the growth of the natural flora and coliform inocula on beef, possibly due to the presence of calcium chloride. Calicium alginate coatings were found to enhance the microbiological safety and quality of poultry products during chilled storage [57], to meats and seafoods [58] and minimally processed fruits. Furthermore, alginate- calcium based edible coating containing Vitamin C and tea polyphenols were used for shelf-life extension of fish [23].
Cross-linked chitosan
Cross-linked chitosan films as coatings for foods including fruits and vegetables can be created by immersion of the food into the acidic chitosan solution. After removing from the chitosan solutions the food are dipped into aqueous glutaraldehydesolution at pH 7 in order to induce crosslinking of the chitosan layer to prevent its leakage easily from the surface [46]. A wide variety of chitosan based antimicrobial films have been well documented [59,60].
Polysaccharide-protein edible coatings
Biopolymers including proteins and polysaccharides have been the focal point of an expanding number of studies reporting their potential use in new materials, such as edible films. One example of proteins is the soy proteins which are used as functional ingredients in food manufacturing because of their role in human nutrition and health. Soy proteins are classified according to their sedimentation properties, into four groups, 2S (8%), 7S (35%), 11S (52%), and 15S (5%). Soy protein is known to be heat-stable due to the extensive disulphide bonds between the subunits. This characteristic limits the use of soy proteins in many applications [61]. Native soy protein, because of its quaternary and compact tertiary structure has limited foaming and emulsifying properties. It consists of 18 different amino acid monomers. Some side chains can interact and/or react with organic or inorganic substances and cellulose fibers. These side chains can be chemically, physically or enzymatically modified to achieve desired properties. The protein molecules dissolve and unfold in solution. The unfolded molecules have an increase surface area, thus there is an increased contact surface area. These unfolded molecules become entangled with each other during curing, which enhances bonding strength. It was proved that the combination of polysaccharide and protein material showed a synergism resulting in a better mechanical properties [62].
Polysaccharides are used in admixture to proteins mainly to enhance stability of dispersed systems. Most high-molecular weight polysaccharides being hydrophilic do not have much of tendency to adsorb at the air–water interface, but they can strongly enhance the stability of protein foams by acting as thickening or gelling agents.
On another hand, citric acid is an important agent for crosslinking, it contains carboxyl groups that may interact with amino groups in soy protein [63]. Also, with sodium hypophosphite (NaH2PO2) as the catalyst, citric acid can interact with the cellulose in soy carbohydrates. A study on protein-polysaccharides interactions showed that blending the anionic quinoa protein with cationic chitosan resulted a monophase film with better mechanical and water-vapor permeability properties from those of pure chitosan. The prepared blend films proposed to be used as edible films for packaging purposes in the food industry [64], while chitosan-whey protein films carrying a high amount of whey protein at acidic pH were found to be biphasic [65]. Whey protein isolate- Pullulan combinations have been investigated throughout a research work. The edible films produced were low solubility and high transparency when compared with synthetic polymer films [66]. Another study showed that the structure and properties of soy protein isolate were improved by blending with carboxymethyl cellulose [67]. New edible coatings based on a mixture of galactomannan–collagen blends have been demonstrated as important tools to extend fruits shelf-life by decrease the fruits gas transfer rates [68].
Research into the manufacture of edible degradable films/ casings using a wide range of food ingredients and extrusion technology has been reported [69]. Films/casings with commercial potential were formed from pectin and gelatin/sodium alginate blends using extrusion technology. Sausages manufactured using casings containing 5% olive oil were found to be more prone to lipid oxidation than those using corn oil [70].
Lipid-Protien edible coatings
Although protein films have a relatively good oxygen barrier and mechanical properties at low and intermediate relative humidity due to their large number of polar groups and extensive polymer interchain, the edible films prepared from protein do not act as an efficient water vapor barrier due to the hydrophilic nature of proteins. In contrast, lipid films have low water vapor permeability owing to their hydrophobic nature but are very brittle because of their monomeric structure. Besides, lipids generally produce opaque films or coatings and are often sensitive to oxidation. These properties may influence the organoleptic attributes of food and lower its marketability.
The above shortcomings can be solved by composite formulations of lipid- protein edible coatings. Various composite films with different formulations were produced had the potential to provide both the relatively high water vapor barrier of lipid films and the desirable mechanical properties of protein films. Novel edible composite films were prepared from pistachio globulin protein, saturated fatty acids, and an emulsifier using the emulsification technique. The resulted emulsified films had improved barrier properties and water solubility. However, the emulsified films were mechanically weaker and less transparent than the control film [71].
The hydrophilic nature of casein and caseinate based films limits their moisture barrier ability when compared to commonly use synthetic plastic films. The addition of calcium caseinate to sodium caseinate–oleic acid–beeswax films showed to improve the mechanical, barrier and optical properties of the films [72].
A mixture of whey protein isolated, glycerol and beeswax was formulated and applied on Chihuahua cheese. The coated cheese samples remain more stable after 10 storage days at 10 ºC under an air velocity of 3m/s. They had a lower moisture reduction and more constant color than the control cheese without coating [73].
Film-forming emulsions were formulated by combining proteins with essential oils. Incorporation of essential oils in protein films gave rise to films with less surface roughness. The use of cinnamon or ginger essential oils at the low ratio used (less than 1:0.1 protein to lipid ratio) in sodium caseinate films showed to slightly reduced water vapour permeability, while their incorporation in the soy protein isolate films had slightly decreased the water vapour barrier efficiency of soy protein isolate films [74,75].
In this work, edible films and coatings, their sources and approaches for their development and utilization are highlighted for the purpose of reservation which was the subject takes more attention in the present review. The review focued on the advances on edible coating compositions and functionalities, besides the trends in the research about their different types, methods of formulations and incorporation of novel technologies to promote their applications for packaging and medical uses as carriers and supports for bioactive molecules.
Bio chemistry
University of Texas Medical Branch, USADepartment of Criminal Justice
Liberty University, USADepartment of Psychiatry
University of Kentucky, USADepartment of Medicine
Gally International Biomedical Research & Consulting LLC, USADepartment of Urbanisation and Agricultural
Montreal university, USAOral & Maxillofacial Pathology
New York University, USAGastroenterology and Hepatology
University of Alabama, UKDepartment of Medicine
Universities of Bradford, UKOncology
Circulogene Theranostics, EnglandRadiation Chemistry
National University of Mexico, USAAnalytical Chemistry
Wentworth Institute of Technology, USAMinimally Invasive Surgery
Mercer University school of Medicine, USAPediatric Dentistry
University of Athens , GreeceThe annual scholar awards from Lupine Publishers honor a selected number Read More...