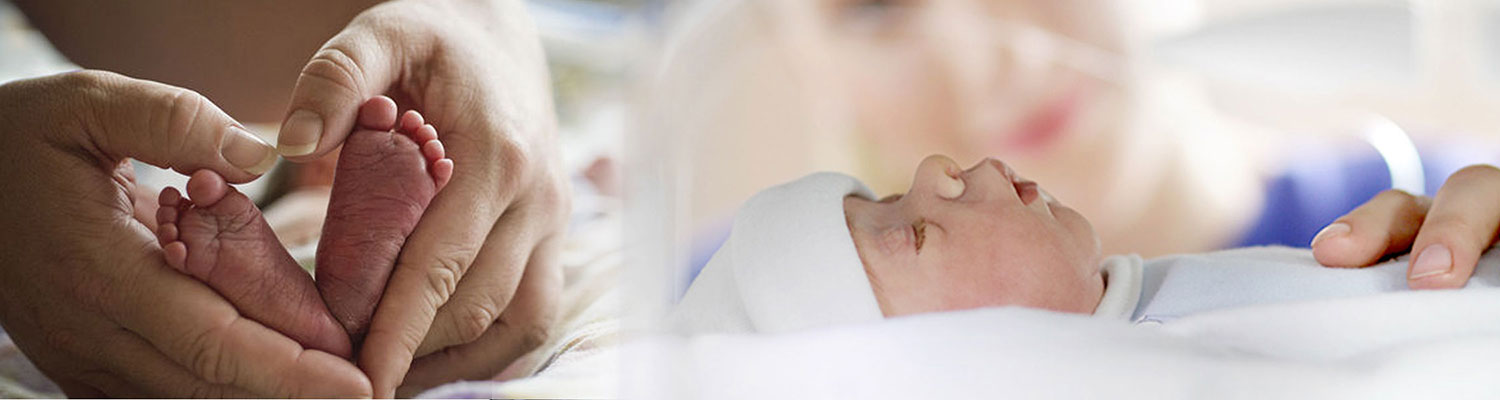
ISSN: 2637-4722
Larissa María Gómez Ruiz1, Norma Guadalupe Ruiz Velazco Sandoval2*, Miriam del Carmen Hernández Dávalos1, Felipe de Jesús Lozano Kasten3, Alejandro Aarón Peregrina Lucano4, Horacio Guzmán Torres3
Received: April 13, 2023 Published: April 20, 2023
Corresponding author: Norma Guadalupe Ruiz Velazco Sandoval, Nursing Department for Care, Development and Preservation of Community Health, CUCS, University of Guadalajara, Mexico
DOI: 10.32474/PAPN.2023.04.000185
Exposure to herbicides in neonates, described in this article, was detected from the realization of a biomonitoring study, which was proposed due to the concern and academic interest on identifying the probable presence of agrochemicals in this population. The sample consisted of 280 neonates from a University Hospital. The determination of herbicides was by high performance liquid chromatography coupled with tandem mass spectrometry (HPLC-MS/MS). Nine herbicides were studied, from which three were found: Molinate in 67 neonates (23.9%), statistical mean of 408, SD 229.3; 2-4D in 64 neonates (22.8%), mean 38, SD 5.6; Glufosinate in 34, (12.1%), mean 575, SD 385.2. None of the mothers reported a history of voluntary exposure to herbicides; the maternal route is the route of exposure. From this type of monitoring, the magnitude of herbicides present in the neonatal population cannot be underestimated, as it becomes important when at least 50% of the world’s population lives in cities and this number is expected to increase to 60% by 2030.
Keywords: Neonates; biomonitoring; herbicide exposure; agrochemicals
Academic and political concern and interest on pesticides in childhood began in the 1990’s, approximately 50 years after the appearance of the book “Pesticides in the Diets of Infants and Children” published by the U.S. National Academy of Sciences. This book explores regulatory approaches that protect infants and children from these agricultural technologies. The authors conclude, 30 years ago, that the health risks experienced by these age groups, as a result of their exposure to dietary pesticide residues, may differ from those experienced by adults, both in nature and severity, since infants and children present a unique susceptibility to the toxic effects of pesticides because they are undergoing rapid tissue growth and development. Traditional approaches to toxicological risk assessment of these agricultural technologies may not always adequately protect children. Although current uncertainty factors used to extrapolate toxicological data to humans provide 10-fold variations between species and within the human population, additional protection for developmental toxicity may be required. The above, of course, depends on the toxicant of interest and the amount of testing that has been performed. It is worth mentioning that socioeconomic, nutritional and health factors influence the vulnerability of pediatrics to toxicants. However, an additional uncertainty factor of up to 10-fold is currently used when prenatal developmental effects are observed [1].
Sixty-five years after the discovery of the first herbicide, Landrigan said that chemical herbicides, as products of the technological development of the companies that produce and market them, represent almost 40% of the world’s pesticide use and their application is increasing. Their main use is in the production of food crops genetically modified to be resistant to glyphosate (Roundup); it should be noted that this herbicide is the most widely used herbicide in the world. Glyphosate-resistant crops, known as “Roundup Ready”, currently account for more than 90% of all corn and soybeans planted in the United States. This herbicide is widely detected in the air and water of agricultural areas, and glyphosate residues are also found in commonly consumed foods. Epidemiological studies in agricultural workers occupationally exposed to glyphosate and other herbicides have shown an increased incidence of non-Hodgkin’s lymphoma on these individuals. Toxicological studies of experimental animals exposed to glyphosate show strong indications of dose-related carcinogenicity in several anatomical sites such as renal tubule carcinoma and hemangiosarcoma. Based on these results, the International Agency for Research on Cancer has determined that glyphosate is a “probable human carcinogen”; a conclusion contested by the manufacturer of glyphosate [2].
Historically, herbicides were developed with the design and introduction of 2,4-D shortly after World War II. Some “natural” methods of weed control, such as salt rocks, have been used since Roman times. The use of synthetic herbicides began around the second half of the 1940s, with wartime research and development of defoliants leading to the release of 2,4-D in 1946. Herbicides have been designed by many companies manufacturing petroleum products, e.g., paint, rubber, and plastics. In the decades following the release of 2,4-D, about 270 active ingredients have been marketed as herbicides. These chemicals represent 17 distinct mechanisms of action, or biochemical sites of action, primarily enzymatic in that their mechanism of action or behavior is through inhibiting physiology in a potentially lethal manner. There is now greater knowledge about the biology of living organisms derived from analytical (combinatorial chemistry, QSARS, metabolomics), biochemical (proteomics), and molecular (genomics, transcriptomics) technologies that are creating an explosion on information about the biology of living organisms. These approaches are being exploited for herbicide discovery and selection, increasing the capacity of chemical syntheses, and facilitating the discovery of herbicides based on their action. The main strategy in herbicide design and production is based on eliminating or reducing the expression level of individual genes, selecting those that create a negative growth phenotype, and then working to identify their function [3].
Agrochemicals were introduced in Mexico with the adoption of the industrial agricultural model in 1947. They were introduced by the Rockefeller Foundation and the Ministry of Agriculture with the objective of transforming Mexican agriculture through agricultural technological innovation. This event was the starting point for the intensive and extensive use of synthetic chemicals for agricultural production. The model consisted of giving exogenous chemical nutrients (granulated fertilizers) to the soil, reducing labor through the use of machinery and controlling weeds with herbicides [4]. Glyphosate-based herbicides were designed to be more precise in pest control and avoid damage to the intended crop, however, they have an environmental impact on water and soil because their permanence is longer than previously known; glyphosate metabolites, for example, are widely present in the world’s soybean supply. On the other hand, it is remarkable that the regulations in the United States of America and the European Union regarding the tolerable daily intake of glyphosate are based on outdated scientific data [5]. In addition, they cause other negative effects such as reduced connectivity (trophic interrelationship between organisms), loss of biodiversity, ecological imbalance and decreased environmental stability. Similarly, the chemical compounds contained in herbicides cause damage to human health such as respiratory disorders, dermatosis and intoxications, the latter occurring through direct use by inhalation, or indirectly through skin absorption [6-8].
In real life, the design of the processes of production, marketing and application of herbicides demanded by the vast majority of processes used in agribusiness are not shared by other scientific disciplines, mainly by the environmental health sciences, since they share very weak analogical arguments, thus turning herbicides into an object of study far from social reality. The logic of agricultural production shapes such production, but outside this logic, it can affect or shape human health and the well-being of children. Therefore, health sciences demand other rigorous knowledge schemes with the objective of knowing and determining, in time, space and place, the presence of herbicides in the prenatal phase of human development. Therefore, biomonitoring becomes important because it uses analytical methods that allow the accurate measurement of low levels of environmental chemicals in human tissues, as they are absorbed into human tissues. [9]. Biomonitoring of pesticides is nowadays frequently performed, mainly in the population working in agriculture [10]. Using such methodological strategies, neonatal herbicide biomonitoring becomes an important epidemiological surveillance tool at this stage of life, as it can demonstrate the route of exposure in the intrauterine developmental stage as background and offers insight for present and future risk assessment purposes.
A cross-sectional and exploratory study was conducted at the Civil Hospital of Guadalajara “Dr. Juan I. Menchaca” with the authorization of its Ethics Committee. Between January 1, 2020, and June 30, 2020 (six months), 1,414 births were attended. To determine an adequate sample size, the formula proposed by Spiegel and Stephens was used [11]. A sample of 280 neonates was obtained. The selection of the study subjects was by consecutive cases, not random. Maternal data such as maternal age, number of gestations, gestational age, occupation, domestic pesticide use, exposure to pesticides during pregnancy, type of pesticide used during pregnancy, frequency of exposure to pesticides, newborn weight, and head circumference were included.
From 3 to 20 ml of urine was taken from neonates in the first 24 hours of life, urines were frozen at minus 12 degrees centigrade, later the samples were transported to the laboratory, then the urine samples were defrosted, 1mL of each sample was taken and deposited in an Eppendorf® 1.5 mL tube, 100 μL of acetonitrile was added, then mixed in a vortex for 1 minute, followed by a 4500 rpm centrifugation at 4°C that lasted 10 minutes, this was realized in a Eppendorf® refrigerated centrifuge; the supernatant was transferred to a Agilent Technologies® 1.5mL vial for its later analysis with an Agilent Technologies Model 1200 equipment for HPLC and model 6430B for tandem mass spectrometry. The HPLC method will use a Zorbaz Eclpse XDB C 18 column, fast resolution, 2.1 x 50 mm, 3.5 μm. Mobile phases: A, 0.1% formic acid in water; B, acetonitrile, 40% to 100% gradient B; injection volume, 5 μL; flow rate, 0.5 min; curve range for each pesticide, 0.01 to 1000 μg/mL.
All the mothers reported no history of voluntary exposure to herbicides or any other pesticide. Occupation showed that they were mainly housewives=250 (89.3%); students= 8 (2.9%); merchants= 8 (2.9%); professionals= 4 (1.4%); secretaries= 2 (0.7%). The median age was 24 years, with a minimum of 14 and a maximum of 41.6. The median number of pregnancies was 2, with a minimum of 1 and a maximum of 8. The median gestation time was 38.4 weeks, with a minimum of 35 and a maximum of 41.6 weeks. Marital status: unmarried= 183 (66%); single= 53 (19%); married= 43 (15%); divorced= 1 (0.4%). Schooling: no schooling= 7 (2.5%); primary or basic= 56 (20%); secondary=131 (46.7%); high school=73 (26%); university= 12 (4.2%); technical studies= 1 (0.35%). Newborn weight had a median of 3,060, minimum of 1,880 and maximum of 4,460 kg. The median head circumference was 34, minimum 30, maximum 38 cm. Regarding herbicides, it was found that, of the 9 herbicides studied, three were found: Molinate in 67 neonates (23.9%), with a mean DE concentration of 408 ± 229.3; 2-4D in 64 neonates (22.8%) mean and DE 38.1± 5.6; Glufosinate in 34, (12.1%) mean and DE 575 ± 385.2 (Table 1).
This exploratory and cross-sectional study yields data showing the presence of herbicides in women and their children who were treated at a university hospital and who reside in a conurbation city of 6 million inhabitants. The presence of herbicides detected leads us to ask whether their presence is due to a significant contamination of the city’s environment (from the application of herbicides in gardens and parks), or from the food that arrives from the countryside to be consumed by all the city’s inhabitants, or both. From these data referred to in this segment of the population, one could assume a magnitude of herbicides present in the population that cannot be underestimated. This becomes important when more than a half of the world’s population lives in cities and is expected to increase to 60% by 2030 [12]. Studies on the presence of herbicides in pregnant women with urban residence are relatively new. Dahiri and Martin-Reina have reported that the application of higher concentrations of herbicides in urban areas is currently gaining importance. Regardless of sex, exposure conditions and environment, they can have different health effects from early life stages, resulting in different detrimental effects on neurodevelopment in newborns and different types of cancer [13]. More recently, Canadian researchers set out to identify urinary concentrations of glyphosate and glufosinate and their metabolites in 2000 pregnant women with predominantly urban residence and found that almost 75% of the women had detectable concentrations of glyphosate, where detectable concentrations of glufosinate were found in between one and six percent of the women. The aforementioned can be considered a public health problem that has been scarcely studied [14]. The three herbicides detected in the neonates (Molinate, 2-4D, Glufosinate) have particular qualities:
a) Molinate belongs to the chemical group: thiocarbamate, classified as II. Moderately hazardous (WHO); III. Slightly toxic (EPA). Its toxic action and symptoms: Topical toxicity: irritant capacity: ocular positive (moderate); dermal positive (moderate); allergenic capacity: nd. Chronic and longterm toxicity: neurotoxicity: nd; teratogenicity: unclear; mutagenicity: nd; carcinogenicity: nd (IARC); suggestive (EPA); endocrine disruption: category 2; other reproductive effects: sperm toxicity; genotoxicity: nd; Parkinson’s: nd; other chronic effects: inhibits thyroid function. Inhibits cholinesterase. EU Risk Phrases: R20/22: Harmful by inhalation and if swallowed. R40: Possible carcinogenic effects. R43: May cause sensitization by skin contact. R48/22: Harmful, danger of serious damage to health by prolonged exposure if swallowed. R63: Possible risk of harm to the unborn child [15].
b) 2-4D, belongs to the chemical group: phenoxyacetic acid, chlorinated: Regarding Human Toxicity; Acute Toxicity. Oral (rats): 469 mg/kg, 639-764 mg/kg; inhalation (rats): 1.79 mg/L; dermal (rats): >1600 mg/kg; dermal (rabbits): >2400 mg/kg. Classification: II. Moderately hazardous (WHO); nd (EPA). Toxic action and symptoms: Topical toxicity: irritancy: eye positive (severe); dermal positive (moderate); allergenicity: positive. Chronic and long-term toxicity: neurotoxicity: level 1; teratogenicity: positive (cleft palate, skeletal malformations); mutagenicity: negative; carcinogenicity: 2B. Possible human carcinogen (IARC); D. Not classifiable as a human carcinogen (EPA); endocrine disruption: category 1; other reproductive effects: decreased sperm count and motility, increased percentage of abnormal sperm in exposed workers; genotoxicity: positive (chromosomal aberrations); Parkinson’s: positive; other chronic effects: bronchitis, peribronchitis, pneumosclerosis, nephrotoxic and hepatotoxic. EU Risk Phrases: R22: Harmful if swallowed. R37: Irritating to respiratory system. R41: Risk of serious damage to eyes. R43: May cause sensitization by skin contact. Known for: Risk of endocrine disruption and soft tissue sarcoma. Known to be one of the components of “Agent Orange” and “Agent White” used in the Vietnam War, and for having dioxin contaminants but not TCDD [16].
c) Glufosinate ammonium (D, L-phosphinothricin or 2-amino-4-(hydroxymethylphosphinylbutanoic acid) has an unusual physicochemical profile compared to most herbicides currently used worldwide, it is the most important herbicide directed against glutamine synthetase (GS). The mode of action of glufosinate has been highly controversial for almost 30 years, since its first introduction in 1993. In addition, environmental conditions can greatly affect glufosinate performance in the field, and only a few weed species have developed resistance to glufosinate worldwide. The toxicity of the active ingredient has been extensively studied. Exposure to high levels of glufosinate can cause a reversible reduction in the concentration of glutamines in mammalian tissues. Subchronic feeding studies of glufosinate were conducted for 3 months at various concentrations using rats (up to 4000 mg kg-1diet), mice (up to 1280 mg kg-1diet) and dogs (up to 256 mg kg-1diet), with no adverse effect levels observed. A chronic toxicity and oncogenicity study showed that at very high concentrations (between 140 and 500 mg kg-1diet), only mild effects were observed on female rat mortality, kidney weight, anemia in both sexes, no neoplastic or non-neoplastic lesions related to glufosinate. Exposure of mice to glufosinate prenatally reduced physical activity, impaired memory formation and autism-like behaviors were detected. This was associated with a significant alteration of the gut microbiome [17].
The approach to the study of prenatal biomonitoring of herbicides and other insecticides is scarce in pediatrics, neonatology and in the field of children’s environmental health. Latin America has a great technological lag, and, despite this, there is an international consensus on the need to produce more knowledge, and even more, to use it as input for public policies to ensure that birth, growth and child development occur in healthy environments [18].
We would like to thank the mothers of the neonates and the staff of the Neonatology Service of the New Civil Hospital of Guadalajara “Dr. Juan I. Menchaca” for all the opportunities to carry out this study.
The authors declare that the research was conducted in the absence of any commercial or financial relationships that could be construed as a potential conflict of interest.
Bio chemistry
University of Texas Medical Branch, USADepartment of Criminal Justice
Liberty University, USADepartment of Psychiatry
University of Kentucky, USADepartment of Medicine
Gally International Biomedical Research & Consulting LLC, USADepartment of Urbanisation and Agricultural
Montreal university, USAOral & Maxillofacial Pathology
New York University, USAGastroenterology and Hepatology
University of Alabama, UKDepartment of Medicine
Universities of Bradford, UKOncology
Circulogene Theranostics, EnglandRadiation Chemistry
National University of Mexico, USAAnalytical Chemistry
Wentworth Institute of Technology, USAMinimally Invasive Surgery
Mercer University school of Medicine, USAPediatric Dentistry
University of Athens , GreeceThe annual scholar awards from Lupine Publishers honor a selected number Read More...