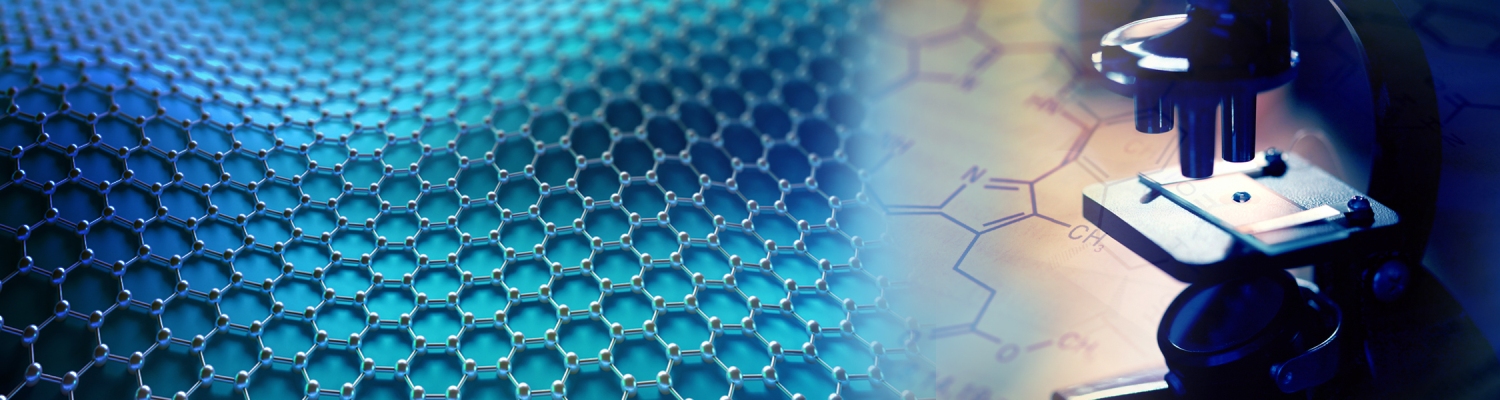
ISSN: 2641-6921
Bahman Zohuri2*, Rahele Zadfathollah Seighalani1
Received:September 16, 2022; Published: November 30, 2022
*Corresponding author:Bahman Zohuri, Galaxy Advanced Engineering, Albuquerque, New Mexico 87111, Chief Executive Officer, USA
DOI: 10.32474/MAMS.2022.05.000207
Fusion energy is widely considered as the ultimate energy of the universe. Fusion nuclear power generation that totally works in opposite direction that of fission nuclear power by splitting atoms nuclei of high Z-materials such as Uranium (U) or Plutonium (Pu), it uses the technology of thermonuclear reaction concepts driven by two isotopes of Hydrogen elements mainly Deuterium (D) and Tritium (T). Recent progress in research towards the development of fusion power driven by means of Magnetic Confinement Fusion (MFC), or high-power energy laser driven Inertial Confinement Fusion (ICF) approach using the same process of D+T fusion as well. MFC is using reactor such as Tokamak device, while ICF is using small droplet of a tiny balloon glass known as pellet in a reactor chamber, where the beams of high-power laser shine on it. Here in this Technical Memorandum (TM), we will be discussing Fusing Energy in a simplified way and Layman Scientific Language that is understandable for public knowledge, which is subject of this Technical Memorandum (TM).
Keywords: Nuclear Fusion Power Reactor, Tokamak Reactor, High Power Laser-Driven Plasma, Inertial Confinement Fusion, Magnetic Confinement Fusion and High-Temperature Environment.
Fusion energy is widely considered as the ultimate terrestrial source of energy very similar to what can be found at the surface of our own galaxy Sun. The goal of the fusion research effort is to derive energy from the nuclear fusion reaction. In this reaction light atomic nuclei such as two isotopes of hydrogen elements namely, Deuterium (D) and Tritium (T), fuse most readily under terrestrial conditions as it is taking place at the surface of our Sun, as illustrated in (Figure 1) In fact, nuclear fusion is an important natural process. Many chemical elements originate from hydrogen through fusion in the process of nucleosynthesis and as we stated, fusion is the energy source of the sun stars. [1] In the process, a Helium nucleus will be produced and it is accompanied by release of a neutron and energy, which in case of D+T fusion process is written as D (2.014102 amu) + T (3.016050 amu) → 2He4 (4.002603 amu) + 0n1 (1.008665 amu) + 17.58 MeV. (Figure 2) [2] Fusion is a form of nuclear energy generated, when light-weight atoms fuse with each other and that is the process at work in every star´s core, releasing an enormous amount of energy. Researchers have been trying to harness fusion and reproduce it on earth in a controlled manner. If they succeed, they will provide the world with a safe, sustainable energy, and environmentally responsible abundant energy source.
However, bear in mind that the energy release per a kilogram of Deuterium is calculated as:
Comparatively, 1 kg of coal produces 28.8MJ; 1 kg of crude oil produces 43.2MJ; and 1 m3 of natural gas produces 37 MJ. [3] Note that one single gram of fusion fuel could generate 90,000 kW. hrs. of energy in a power plant; equivalent to the combustion heat of 11 metric tons or 11,000,000 grams of coal. For decades, the scientific community has been pursuing nuclear fusion, yet now research has reached a critical stage, as scientists are building an experimental reactor that one day may demonstrate that fusion can be used commercially to create electrical power. [4].
Figure 2: Depiction of all Isotopes Hydrogen Thermonuclear Reactions (Source: U.S. Department of Energy)
For more than 50 years, energy has been generated in nuclear power plants through fission, a process in which heavy elements such as uranium are bombarded by neutrons releasing heat in the process. Nuclear fusion, on the other hand, is based on the opposite principle. In fusion reactors, light atomic nuclei are compressed under intense pressure and heat to form heavier ones and release energy in the process. The process must be optimized to generate more energy than it consumes. With a sufficiently large and sustainable energy "profit", fusion could be utilized to generate electricity commercially. The main fuels used in nuclear fusion are deuterium and tritium, both heavy isotopes of hydrogen. Deuterium constitutes a tiny fraction of natural hydrogen, only 0,0153%, and can be extracted inexpensively from seawater. Tritium can be made from lithium, which is also abundant in nature. The amount of deuterium present in one liter of water can in theory produce as much energy as the combustion of 300 liters of oil. This means that there is enough deuterium in the oceans to meet human energy needs for millions of years.
Building a fusion power plant withstanding the immense temperature and pressures produces is one of the century´s greatest engineering challenges. The fuel, made up of the hydrogen isotopes deuterium and tritium, must be heated to about 100 million degrees centigrade. At that hotter-than-the-sun temperature, a fully ionized gas-plasma is formed. The plasma will then be ignited to create fusion. At present, scientists are pursuing two methods for achieving nuclear fusion: inertial and magnetic confinement. Materials that can be used for building fusion reactors are well defined and described in a published form by the authors and for the reader with more interest we refer them to Reference [5] In inertial confinement systems, ion beams or laser beams are used to compress a pea-sized deuterium-tritium fuel pellet to extremely high densities. When a critical point is reached, the pellet is ignited through shock wave heating. Fusion power plants using this technique would ignite fuel pellets several times per second. The resulting heat is then used to generate steam that powers electricity-generating turbines. In magnetic confinement systems, electromagnets are used to contain the plasma fuel. One of the most promising options, the tokamak device, contains the plasma in a doughnut-shaped chamber. A powerful electric current is induced in the plasma, resulting in an increase in temperature. The plasma is also heated by auxiliary systems such as microwaves, radio waves or accelerated particles. In the process, temperatures of several hundred million degrees centigrade are achieved. In the following two sections we define and explain both types of confinements, namely Magnetic Confinement Fusion (MCF) and Inertial Confinement Fusion (ICF).
Magnetic confinement fusion is an approach to generating thermonuclear fusion power that uses magnetic fields to confine fusion fuel in the form of a plasma. Magnetic confinement is one of two major branches of fusion energy research, along with inertial confinement fusion. The magnetic approach began in the 1940s and absorbed the majority of subsequent development. See Figure 3, where hot plasma, magnetically confined in a Tokamak and it is this subject of this TM by introducing Magnetic Confinement Fusion process. Fusion reactions combine light atomic nuclei such as hydrogen to form heavier ones such as helium, producing energy. In order to overcome the electrostatic repulsion between the nuclei, they must have a temperature of tens of millions of degrees, creating a plasma. In addition, the plasma must be contained at a sufficient density for an adequate period of time, as specified by the Lawson criterion (triple product). [6] Magnetic confinement fusion attempts to use the electrical conductivity of the plasma to contain it through interaction with magnetic fields i.e., (Figure 4). The magnetic pressure offsets the plasma pressure. Developing a suitable arrangement of fields that contain the fuel without excessive turbulence or leaking is the primary challenge of this technology. The development of magnetic fusion energy (MFE) came in three distinct phases. In the 1950s it was believed MFE would be relatively easy to achieve, setting off a race to build a suitable machine. By the late 1950s, it was clear that plasma turbulence and instabilities were problematic, and during the 1960s, "the doldrums", the effort turned to a better understanding of plasma physics. In 1968, a Soviet team invented the tokamak magnetic confinement device, which demonstrated performance ten times better than alternatives and became the preferred approach.
Construction of a 500-MW power generating fusion plant using this design, the International Thermonuclear Experimental Reactor (ITER), began in France in 2007. Its most recent schedule is for it to begin operation in 2025. In summary, putting fusion driven plasma energy for producing electricity in a perspective, we may say that our modern society requires environmentally friendly solutions for energy production as a source of generating electricity that is also driven by growth in population globally. Naturally, we are constantly looking for sources of energy, either renewable or non-renewable, that are free of producing any carbon released into its surroundings. In the case of nuclear energy that arguably considered as renewable source of energy, can be released not only from the fission of heavy nuclei, but also from the fusion of light nuclei as it was described at introductory of this technical memorandum as well. Nuclear fusion is an important option for a clean and safe solution for our long-term energy needs, once we could manage to satisfy Lawson Criteria and physics of break-even (i.e., amount of energy that we need to put in for fusing of the two light nuclei of hydrogen isotopes, at least is equal to the amount of energy out from it as it is shown in Equation-1 and (Figure 2) [1, 6] in respect to fusion reaction. The extremely high temperatures required for the fusion reaction are routinely realized in several magnetic-fusion machines. Since the early 1990s, up to 16 MW of fusion power has been released in pulses of a few seconds, corresponding to a power multiplication close to break-even. Our understanding of the very complex behavior of magnetized plasma at temperatures between 150 and 200 million 0C surrounded by cold walls has also advanced substantially. This steady progress has resulted in the construction of ITER, a fusion device with a planned fusion power output of 500 MW in pulses of 400 s as demonstrated in (Figure 5).
The world’s most advanced and largest fusion experiment is the International Thermonuclear Experimental Reactor (ITER) project, with seven international members (China, India, Japan, South Korea, the European Union, the Russian Federation and the United States). Based on the tokamak concept (using a device that confines plasma through a magnetic field), ITER is currently being built in Cadarache, France. As illustrated in Figure 5 the Reactor is designed to achieve a fusion power gain of at least 10 and produce 500 Megawatt (MW) of fusion power. It will also test key technologies necessary for a fusion reactor.
For the past half-century, experimentalists have tried to control the nuclear fusion process in an effort to create a copious and near-limitless source of clean energy. Deuterium (D) exists abundantly in water and Tritium (T) readily produces from lithium. At present, 1012 tons of deuterium can be extracted from the surface water of the earth. Furthermore, unlike fission reactors, fusion reactors produce little and short-lasting radioactive waste. The requisite environmental conditions of fusion, however, unsurprisingly make taming the process a nearly impossible task. The Lawson Criterion strictly defines such a minimum condition for a nuclear fusion device to work. In order to reach ignition, a fusion device must exceed a determined triple product of plasma electron density, plasma temperature, and the rate of energy loss.
To be self-sustaining, this specific threshold is 3 x 1021 m-3 keV s-1. [1, 6] Beyond satisfying conditions, the nuclear reactor must also generate energy.
The Q-factor, reflecting this merit, is defined as the ratio:
If a particular nuclear fusion device fails to exceed a Q-factor of 1, then the device does not possesses a practical value. More importantly, the ratio of electric energy output to input, Q' can be expressed approximately as 1/4(1+Q). This effective break-even ratio fixes the desired Q value to be at least 2 [7]. For a deuterium-tritium reaction to release energy at a level of practical use, the gas must be heated to nearly 100 million Kelvin. At such high temperatures, the plasmatic deuterium and tritium particles move erratically, and most materials fail to confine the ionized gas [5]. The primary solution to confine the plasma for fusion has been to produce a magnetic field. (Figure 4) Even still, the confinement lasts only a few seconds as the instability of the plasma accrues.
The magnetic confinement driven fusion of thermonuclear plasma requires intense magnetic fields as it can be observed in technology of Tokamak machine and its present and modern technology being utilized in ITER by augmenting superconducting magnets for fusion as a newly clean energy with a common goal to decarbonize energy generation, while maintaining reliability and low-cost service to produce electricity to growing demand by end user cliental. See (Figure 7), which shows an overview of the magnetic field system equipping the ITER reactor. The production of these magnetic fields in the large vacuum chamber (i.e., 837 m3) of the International Thermonuclear Experimental Reactor (ITER), currently being built at the Cadarache French site in the Bouches-du-Rhá½¹ne is in itself a major technological challenge. The ITER’s magnetic field system comprises three giant superconducting system as:
a) The Toroidal magnetic Field (TF),
b) The Poloidal magnetic Field (PF), and
c) The Central Solenoid (CS) where, focus on Superconductivity and Superconductors.
These could be said to represent the backbone of the newly designed and innovative approach ITER Tokamak reactor as it is artistically drawn in (Figure 8).
Figure 8 shows the three main superconducting magnetic field systems equipping the ITER experimental reactor. The eighteen Toroidal Field (TF) coils system wound around the torus confines the plasma. The Central Solenoid (CS) system creates a very high current that heats the plasma as it circulates through it, thus generating another magnetic field that contributes to the confinement. The six Poloidal Field (PF) coils system wound around the torus stabilizes the plasma accordingly. As far as integration and augmentation of superconductivity for fusion applications are concerned, from the history of magnetic confinement driven fusion, we know that up to the early 1980s, all magnetic confinement machines or suggested reactors relied on resistive magnets, which were generally built using silver-doped copper in order to improve their mechanical properties. The machines’ small size and, in particular, the fact that they operated in a pulsed regime guaranteed the viability of this solution which was pushed to its limits with the European tokamak of the Joint European Torus (JET) as illustrated in Figure-6. At present, the total energy required to power the JET’s magnets, which is above 1GW, can only be delivered to the machine via energy storage flywheels due to the short duration of the plasma discharge (i.e., 10 to 30 seconds), where discharge plasma indicates the presence of a plasma within the confinement chamber. However, it is obvious that the resistive magnet solution cannot effectively be applied to the continuous operation reactor. This is what drives Europe to extend its fusion program to include the development of the first machine to be powered by superconducting magnets that we know it as tokamak formerly Tore Supra at the CEA center in Cadarache, which has a major plasma radius R of 2.5 m as depicted in Figure 9 here. The technical specification of Tore Supa is as follows:
a) Major radius: 5 m
b) Minor radius: 50 m
c) Magnetic field: 3.7 T (toroidal)
d) Heating power: 17 MW
Again, this machine is tokamak type reactor and started its operation since 2016 up to now and located in Cadarache, France.
Matter and energy are fundamental components of our physical world. These components manifest themselves in a variety of ways under different physical conditions and can be affected by variety of processes. Inertial confinement fusion (ICF) is another way to confine the fourth state of matter that is known as plasma, by means high-power laser driven fusion of D + T. Inertial confinement fusion (ICF) is a fusion energy research program that initiates nuclear fusion reactions by compressing and heating targets filled with thermonuclear fuel. In modern machines, the targets are small spherical pellets about the size of a pinhead typically containing a mixture of about 10 milligrams of deuterium 2H and tritium 3H. Figure 9 shows, the schematic of the different stages of ICF using laser. The Blue arrows represent radiation; the Orange is blowoff, and the Yellow color is inwardly transported thermal energy.
As it can be seen from Figure 10, the following stages are taking place in an ICF process as: [8]
a) Laser beams or laser-produced X-rays rapidly heat the surface of the fusion target, forming a surrounding plasma envelope.
b) Fuel is compressed by the rocket-like blowoff of the hot surface material.
c) During the final part of the capsule implosion, the fuel core reaches 20 times the density of lead and ignites at 100,000,000 0
d) Thermonuclear burn spreads rapidly through the compressed fuel, yielding many times the input energy.
Heating concepts and analysis laser driven fusion and its early calculations suggested that the amount of energy needed to start the propagating burn would be very small; only a tiny amount of fuel had to be raised to the required temperature. This has proven difficult and new methods have emerged in an effort to make this process more efficient. The initial solution to the heating problem involved the careful "shaping" of the energy delivery. The idea was to use an initial lower-energy pulse to vaporize the capsule and cause compression, and then a very short, very powerful pulse near the end of the compression cycle. The goal is to launch a shock wave into the compressed fuel that travels inward to the center. When it reaches the center, it meets the same wave travelling in from the other sides. This causes a brief period where the density in the center reaches much higher values, over 800 g/cm3. [9]
The potential advantages of nuclear fusion energy are manifold, as it represents a long-term, sustainable, economic and safe energy source for electricity generation. Fusion reaction fuel is inexpensive and abundant in nature, while the amount of long-lived radioactive waste and greenhouse gases produced through fusion are minimal. While research on nuclear fusion continues, many spin-offs relating to plasma physics and fusion technology are already benefiting society. These include improvements in materials research, such as ceramic, metals and coatings, and industrial processes such as welding and waste removal [4].
Nuclear energy can also be produced by fusion reactions of light nuclei. This technique promises many advantages and has attracted global research and development efforts. The IAEA has supported fusion energy research since its inception and helps Member States exchange and build knowledge on fusion science and technology. Fusion research and development has continually thrown up new challenges to test the ingenuity and skills of at least two generations of scientists and engineers. There are many challenges still to be faced, and there may be some which are unseen from today’s perspective. Changes across the utility are accelerating, from customer behavior to energy sources to climate risk. We use advanced data modeling and innovative technology solutions to help utilities evaluate the full impact of these interconnected changes—and unlock the possibilities of revenue growth, sustainability, and better customer and community outcomes. Looking into Fusion aspect of Nuclear Energy as the utmost source of clean energy as well as a universal energy, we can easily find it in the Sun and Stars. It also, seems, beside Nuclear Fission energy, the way we need to go in near future is Nuclear Fusion Energy, so long as we can overcome all the related technical issues. This kind of nuclear energy with either the approach of Magnetic Confinement Fusion (MCF) or Inertial Confinement Fusion (ICF), is by all means a clean source of energy that can be produced without any doubts.
Bio chemistry
University of Texas Medical Branch, USADepartment of Criminal Justice
Liberty University, USADepartment of Psychiatry
University of Kentucky, USADepartment of Medicine
Gally International Biomedical Research & Consulting LLC, USADepartment of Urbanisation and Agricultural
Montreal university, USAOral & Maxillofacial Pathology
New York University, USAGastroenterology and Hepatology
University of Alabama, UKDepartment of Medicine
Universities of Bradford, UKOncology
Circulogene Theranostics, EnglandRadiation Chemistry
National University of Mexico, USAAnalytical Chemistry
Wentworth Institute of Technology, USAMinimally Invasive Surgery
Mercer University school of Medicine, USAPediatric Dentistry
University of Athens , GreeceThe annual scholar awards from Lupine Publishers honor a selected number Read More...