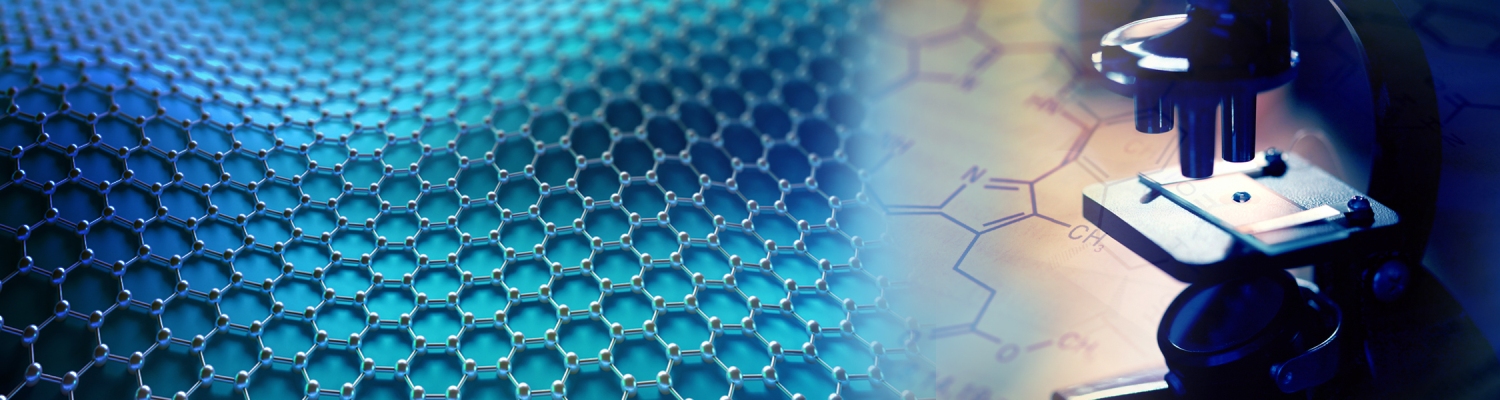
ISSN: 2641-6921
Bianca Pizzorno Backx*
Received: August 12, 2020; Published:September 03, 2020
*Corresponding author: Bianca Pizzorno Backx. UNIVERSIDADE FEDERAL DO RIO DE JANEIRO, Campus Duque de Caxias Professor Geraldo Cidade, Rodovia Washington Luiz (BR-040) Km 104,5 - Santa Cruz da Serra, Duque de Caxias / RJ, Brazil
DOI: 10.32474/MAMS.2020.03.000159
A threat is taking over the world today. SARS-CoV-2 is a virus that spread throughout the planet, behaviorally changing world society. Humanity seeks alternatives to increase the physical barrier associated with the protection of homemade masks.Viruses, which have a semantic origin in the Latin, “toxin” or “poisonous”, are infectious agents that mostly assume a nanometric scale, with a size around 20-300 nm in diameter.An abiotic material, capable of inhibiting the spread of viruses is indispensable. Understanding the virus’s adhesion to the surface of the textile is very important for the choice of the best tissue, which has less adhesion of the virus to the surface. This minimization of virus adhesion can be promoted by the modification of surface characteristics of the textiles. The addition of nanostructures is capable of presenting antimicrobial activity, an essential factor for obtaining efficient textiles for making homemade masks.
Keywords:Homemade mask, Microorganisms, Textiles, Covid-19, Supramolecular interactions
The nanometer is equivalent to 1 billionth of a meter, 10-9m.
The formation of nanoparticles occurs with a large proportion
of their atoms found on the surface compared to the innermost
layers. Nanoparticles have a larger surface area per unit mass
compared to larger particles [1]. Since chemical reactions occur on
surfaces, it means that nanoparticles are extremely more reactive.
Electrostatic stabilization occurs when the repulsive forces exceed
Van der Waals forces [2]. This understanding is based on the forces
of supramolecular interactions [3]. The viral action of nanoparticles
has been widely explored. Silver nanoparticles, for example, have
broad antiviral effects for herpes simplex viruses (HSV), human
immunodeficiency viruses (HIV), Hepatitis B virus (HBV), among
others [4]. AgNPs have efficient, eco-friendly synthesis routes.
The antiviral properties of nanoparticles can involve interaction
with nucleic acids or thiol groups of proteins. For metallic
nanoparticles with antimicrobial action, already established in the
literature, such as, for example, silver nanoparticles (AgNPs), gold
nanoparticles (AuNPs), zinc oxide nanoparticles (ZnNPs), oxide
copper nanoparticles (Cu2ONPs) have different synthesis routes
[5]. Bacteria, fungi, and viruses are of concern to society around
the world. Many studies are based on the ability of new materials
to have an antimicrobial action. Supramolecular systems are
nanostructured organizations able to act between nanostructures
and microorganisms. It can be possible due to their high reactivity
and equivalent size [6]. The SARS-CoV-2 viral particle size is
around 70 to 90 nm [7]. Interaction between nanoparticles and
viral particles is efficient because, through efficient interactions,
nanostructures’ action is efficient. The interaction between
the virus and the adhesion surface is based on intermolecular
interactions [8]. The nanoparticles synthesized by the green route
have interactions between the dispersive medium.
Supramolecular chemistry is the basis of green systems. Able to
stabilize nanostructures in extracts of plant origin. The search for
antimicrobial action associated with nanoparticles is an essential
initiative associated with the low cost, low concentration of
precursor, and almost no environmental impact. Microorganisms, such as viruses, fungi, and bacteria, are often pathogenic and can
cause severe infections in humans, animals, or plants. Thus, there
is a growing need to seek new antimicrobial agents of natural
and non-toxic origin [9]. Viruses do not have an autonomous life,
requiring host cells to replicate their genetic material, RNA, or
DNA and guarantee their survival by producing new viral particles
[10]. Studies at the University of Southampton, England, revealed
the virus’s surface characteristics that caused COVID-19. There
are many proteins on its surface, which are used to connect and
penetrate our cells. In general, they are disguised with sugar-coated
spikes, known as glycans, that help the virus escape the human
immune system [11]. Associating the understanding of adhesion of
the viral particle to different textiles is a primary focus of study. Due
to the immense variation in the composition of the fabrics, some
are mostly composed of natural fibers. Others are compositions
of natural and synthetic fibers, and others called non-woven
fabrics are formed by synthetic fibers chemical binders bond the
fibers or non-woven filaments. For such a wide range of textile
compositions, understanding the supramolecular interactions
capable of increasing virus adhesion or even repelling them is
essential. Understanding whether the viral particle will have more
exceptional adhesion to natural, synthetic fiber fabrics and whether
this adhesion is permanent is of great interest. There is a possibility
that a textile composition is sufficiently capable of repelling the
virus. In cases where the virus tends to adhere, having textile with
nanostructures capable of making it antimicrobial is of immense
advantage [12-16].
Studies should be developed to understand the virus and textiles’ adhesion. It is essential to understand the protection of physical barriers associated with the textiles’ interstice sizes and hydrophobicity of the surface of textiles. The adhesion of viral particles also can be minimized by the quality of the textiles’ fiber. Besides, antimicrobial textiles with the addition of nanoparticles that have antimicrobial activity should be extensively studied to be potentially used to minimize contamination by the virus that causes COVID-19 and other diseases associated with microorganisms.
Bio chemistry
University of Texas Medical Branch, USADepartment of Criminal Justice
Liberty University, USADepartment of Psychiatry
University of Kentucky, USADepartment of Medicine
Gally International Biomedical Research & Consulting LLC, USADepartment of Urbanisation and Agricultural
Montreal university, USAOral & Maxillofacial Pathology
New York University, USAGastroenterology and Hepatology
University of Alabama, UKDepartment of Medicine
Universities of Bradford, UKOncology
Circulogene Theranostics, EnglandRadiation Chemistry
National University of Mexico, USAAnalytical Chemistry
Wentworth Institute of Technology, USAMinimally Invasive Surgery
Mercer University school of Medicine, USAPediatric Dentistry
University of Athens , GreeceThe annual scholar awards from Lupine Publishers honor a selected number Read More...