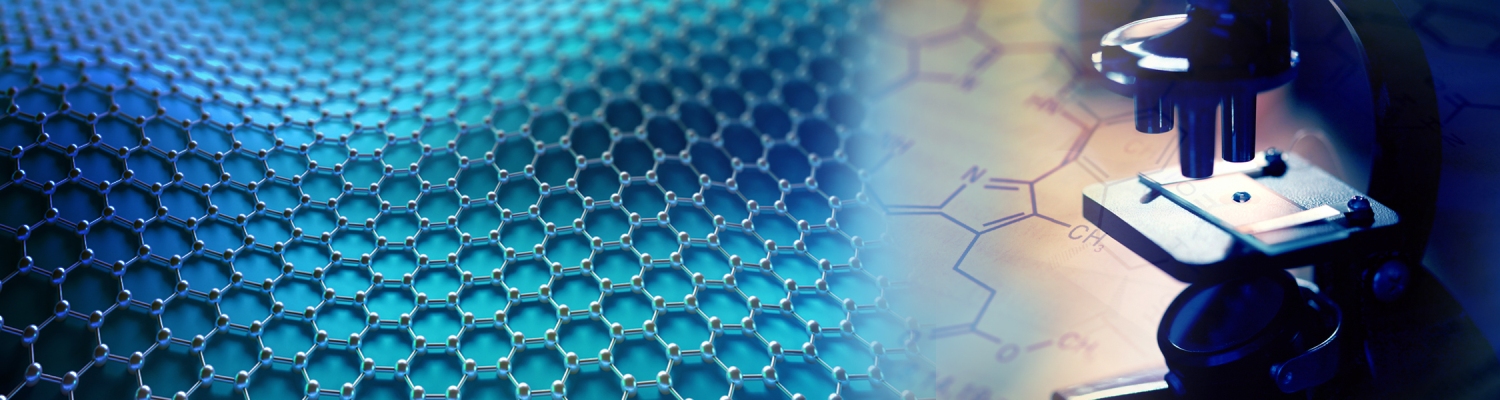
ISSN: 2641-6921
Ali Hilal-Alnaqbi1* and Sawsan Dagher2
Received: November 15, 2019; Published: December 02, 2019
*Corresponding author: Ali Hilal-ALNAQBI, Abu Dhabi Polytechnic, Abu Dhabi, UAE, Email ID:Ali.Alnaqbi@adpoly.ac.ae
DOI: 10.32474/MAMS.2019.02.000135
Smart materials are a group of materials that alter its properties based on changes in their environment, thus making them stimuli-responsive materials. This study provides an overview of the six main categories of the recently used smart materials, namely, piezoelectric materials, photovoltaic materials, shape-memory materials, magneto strictive materials, thermoelectric materials, and chromogenic materials.
Keywords: Piezoelectric; Photovoltaic; Shape-Memory; Magneto strictive; Chromogenic
Smart materials play a huge role in new technologies, which was
recently addressed by several reports. Smart materials and systems
will be specifically, critical to the enhancement of quality of life,
economic welfare, productivity, and sustainability. The adoption of
smart materials is associated with other rapidly rising technologies,
including nanotechnology, microelectronics, biotechnology, new
manufacturing processes, and robotics. Nowadays, companies
and commercial sectors have been utilizing smart technologies
in many of their products. The construction sector, being a
huge contributor to sustainability and energy consumption, has
improved its performance since implementing some of these
products; self-cleaning materials, self-healing constituents, anticorrosion
coatings, and smart windows. Furthermore, smart
technologies have been utilized for biomedical applications such as
smart drug delivery, antibacterial treatments, and cellular repair.
Smart materials are high-performance engineering materials, in
which one of their properties can be altered in a predictable or
controllable manner such as
(i) Mechanical Properties (E.G. Shape, Stiffness, Viscosity)
(ii) Thermal Properties
(iii) Optical Properties
(iv) Electromagnetic Properties
These properties can be changed by an external stimulus or
environment such as temperature, pressure, light, laser heating,
radiation, microwaves, voltage, electricity, pH, moisture, chemicals,
magnetic field, or mechanical force. Smart materials revert to their
original states once the external stimulus is removed, since the
change is reversible and can be repeated many times [1].
Recently a wide variety of smart materials are developed and can be utilized in numerous scientific fields. Each has different properties that can be changed for specific applications. The common emerging classes of smart materials are shown in (Figure 1) as following: Piezoelectric materials generate a voltage when stress is applied. Piezoelectric effect is reversible, in which a stress will be produced when an electric field is applied. The most common piezoelectric material is a single crystal quartz. Other materials are PZT, lithium tantalate, lead titanate ceramic, barium titanate, lithium niobate, and piezoelectric polymers. Polymers have lower piezoelectric constant than ceramics, thus the shape change of piezoelectric polymers is less than that of piezoelectric ceramics considering the same amount of voltage applied. Also, ZnO thin film is extensively used in piezoelectric devices for acoustic wave’s generation, due to the fact that ZnO has a large electromechanical coupling [2]. Photovoltaic materials have the ability to convert light into electricity, known as photoelectric effect, using semiconducting materials such as silicon, gallium arsenide and nanomaterials (e.g. ZnO, TiO2, CuO, PbS). Photovoltaic materials absorb photons of light. As the photons’ energy equal to or greater than the band gap of the material electrons can be excited, creating mobile electrons and holes, these free electrons generate an electric current that can be used as electricity [3-10].
Shape-memory materials are stimulus-responsive materials that can be actuated on demand to recover their original shape, after being quasi-plastically distorted. Shape memory materials are ideal for an integrated intelligent system, in which the structure can sense the environmental change and then generate reactive motion as pre-programmed. The most common shape-memory materials are shape-memory alloys (SMA) and shape-memory polymers (SMP), in which a deformation in shape or size is obtained in response to temperature changes or stress changes (pseudoplasticity). Furthermore, magnetic shape memory alloys can be stimulated by applying a magnetic field to change their shape. The basic concept of the SMA working mechanism, that it will recover to its original form when heated beyond a certain temperature (i.e., the transformation is reversible), and the transformation in both directions is instantaneous. To fix the original “parent shape,” the alloy must be held in position and heated to about 500°C (932°F). This process is usually called shape setting. The actuation temperature can be determined accurately based on the SMA composition. SMA are a group of metallic alloys such as nitinol (TiNi) which, as previously mentioned, can return to their original shape and size when subjected to a memorization process between two transformation phases (martensite and austenite phases), which is temperature dependent. The martensite structure is stable at low temperatures and the austenite structure is stable at higher temperature. When a SMA is heated, it begins to transform from martensite into the austenite phase. This transformation phenomenon is known as the shape memory effect (SME). SMA exhibits large stress and strain factors, long fatigue life, and chemical corrosion resistance. SMP are distinguished from SMA by its unique properties such as light weight, ease of processing, high shape recovery of up to 400% plastic strain, chemical stability, transparency and low cost. Consequently, SMP are widely used for aerospace applications such as light actuators, structural parts with reduced size during transport, and expandable/deployable structures [11-13].
Magneto strictive materials change their shape (expand or contract) under the influence of magnetic field. Because of the rotation and alignment of the molecular dipoles and magnetic field boundaries of the material as a response to a magnetic field. This causes the material to strain and elongate, and vice versa, adjust their magnetization when mechanical stress is applied. Ferromagnetic materials are the most effective magnetostricive materials, due to the fact that they are isotropic with few impurities, which allows their molecular dipoles to rotate easily, and can be used to produce vibrations. As the applied magnetic field increases in intensity, the magneto strictive strain on the material increases. Magneto strictive materials include nickel, Co-Ni, Fe-Al, Fe-Ni (Permalloy), Fe-Co, Co- Fe-V, CoFe2O4 and NiFe2O4, and some rare earths and their alloys and compounds. Magneto strictive materials are used in several applications including actuators and sensors, ultrasonic cleaning devices medical devices, underwater sonar, industrial vibrators, vibration, and noise control systems [14]. Thermoelectric materials can directly convert heat into electricity and vice versa, based on See beck and Peltier effect. Thermoelectric materials utilize lost thermal energy or waste heat into efficient electricity for cooling applications. The most commonly used thermoelectric material is based on bismuth telluride (Bi2Te3). A typical thermoelectric material should have high electrical conductivity and low thermal conductivity such as Bi2Te3 and PdTe with piezoelectric impedance (ZT) about 1. However, these materials have some drawbacks, such as high cost, low stability at high temperatures, and environmental concerns. As an alternative, currently, intensive research is going on developing nanostructured thermoelectric materials. Thermoelectric materials are utilized in optoelectronic devices, solid state thermoelectric cooling, biothermal batteries, space applications, cooling devices and automotive power generation. Nevertheless, further improvements are required for optimization of both materials and devices’ performance [15-18].
Moreover, chromogenic materials are materials that change their color as a response to external stimuli (changes in environmental condition) this property is utilized in a wide range of applications. Four types of chromogenic materials are available based on the external stimuli that triggers the change in its color, these four types of chromogenic materials are
a. Photochromic materials, exhibit a change in color in response
to light, (e.g., light-sensitive sunglasses in which their color
become darker in the presence of bright sunlight)
b. Thermochromic materials show alteration in color according
to the change in their temperature
c. Electrochromic materials which change their opacity when a
voltage is applied (e.g., liquid crystal displays)
d. Halochromic materials change their color as a result of acidity
change
One application of halochromic materials is in paints for metal coating, thus corrosion in the metal can be indicated by the change in the color of the paint layer covering the metal. The process in which these materials change color is named chromism. In principle, the stimulus alters the equilibrium of electrons inside the molecule, which further leads to breaking the chemical bonds in the Chromogenic material. Consequently, altering some of the materials’ optical properties, such as absorption, transmission, reflectance, or emission. When the stimulus terminates, the material returns to its original electronic state, retrieviving its original optical properties (i.e., initial color or transparency) [19].
Smart materials are key to future solutions in technological advancements, impacting distinct fields, such as, pharmacy, engineering, biology, chemistry, architecture, automotive industry, and communications. The technology of smart materials is significantly impacting modernity and civilization, nowadays, it is the most auspicious technology that expand efficiency and save resources by swiftly reacting to corrosion, pH changes, water content, temperature, mechanical forces, and many other. This can outspread the service life of components and structures, reducing life cycle costs of structures including inspection, maintenance, and repair costs. Wisely acknowledging, understanding, and monitoring the configuration and microstructure of any new material is the key to enhancing and developing a smart material. Modern and advanced smart materials will unquestionably enhance the quality of our life.
Bio chemistry
University of Texas Medical Branch, USADepartment of Criminal Justice
Liberty University, USADepartment of Psychiatry
University of Kentucky, USADepartment of Medicine
Gally International Biomedical Research & Consulting LLC, USADepartment of Urbanisation and Agricultural
Montreal university, USAOral & Maxillofacial Pathology
New York University, USAGastroenterology and Hepatology
University of Alabama, UKDepartment of Medicine
Universities of Bradford, UKOncology
Circulogene Theranostics, EnglandRadiation Chemistry
National University of Mexico, USAAnalytical Chemistry
Wentworth Institute of Technology, USAMinimally Invasive Surgery
Mercer University school of Medicine, USAPediatric Dentistry
University of Athens , GreeceThe annual scholar awards from Lupine Publishers honor a selected number Read More...