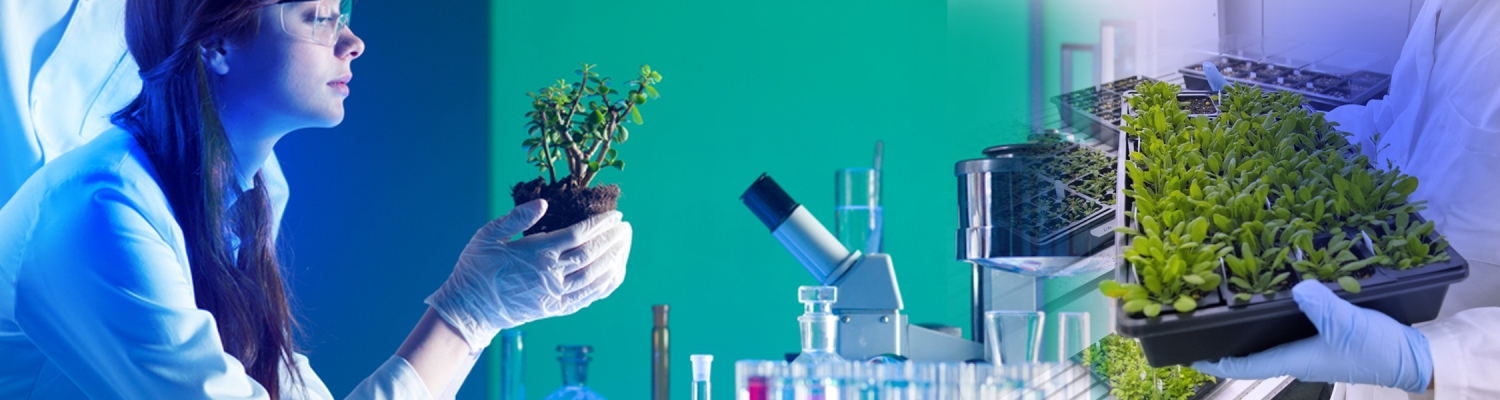
ISSN: 2641-6794
Yousef Najjar*
Received: June 17, 2019; Published: June 28, 2019
Corresponding author: Yousef Najjar, Department of Mechanical Engineering, Jordan University of Science and Technology, Irbid, Jordan
DOI: 10.32474/OAJESS.2019.03.000154
Civilization relies on two basic commodities: water and energy. Potable water is a scarce critical resource. It is the lifeblood for everything living on earth Water and energy very much rely on each other. Without both of them in ample supply, all else fails. Water is considered as a very important part of energy generation and development. There are many utilizations for water such as using it directly in hydroelectric power generation, and it used also in cooling thermal power plant plus controlling the air emissions. One more utilization of water is in extracting, refining and processing energy resources. There are a new technology needed to make the energy and water more useful in permanent future because the problems of supplying water are not serious only for the arid regions but have become a global issue.
Keywords: Energy; Water; Distribution; Water Consumption; Extracted Water; Desalination; Water Treatment; Energy Resource Extraction
Abbreviations: CO: Carbon Monoxide; CO2 : Carbon Dioxide; CSP: Concentrated Solar Power; ED: Electro-dialysis; EDR: Electrodialysis Reversal; EIA: Energy Information Administration; EOR: Enhance Oil Recovery; GE: Geothermal Energy; HF: Hydraulic fracturing; MED: Multi-effect distillation; MSF: Multi-stage flash; MVC: Mechanical vapor compression; NO2 : Nitrous Oxide; PP: Power Plant; PV: Solar Photovoltaic; PW: Produced Water; PWI: Produced Water Intensity; RE: Renewable Energy; RO: Reverse Osmosis; SE: Solar Energy; SO2 : Sulfur Dioxide; TDS: Total Dissolved Solids; TVS: Thermo Vapor Compression; VC: Vapor Compression; WOR: Water to Oil Ratio; WUI: Water Use Intensity
In one hand the water is used to mine, convert and refine the energy resources and in the other hand the fresh water was collected, treated and distributed by using energy, so there are a wide technologies and activities depending on water and energy, such as the cooling of power plants, generating energy from water, desalination, treatment of water and wastewater and reusing water [1-4]. There are many utilizations for energy in our life like generating steam in the power plants, treating water and waste water, heating and cooling buildings [5-9]. In power plants a huge part of water is used for once-through cooling because the cooling water will be returned to the water source at a little high temperature and that is shown in Figure 1 [10].
A many researches to use the energy and water resources with more reliability and sustainability are needed and these researches also are needed to decrease the problems of development between these two important resources, so because that the global consumption of energy will reach 50% by 2030, the demand of water resources and its supplying will be important to support this increasing. As a result, for that the competition between the energy field and water users for already limited fresh water resources in many places in the world will be increased [11-13]. The worries about demanding water for energy and the expected influences to happen on the availability, reliability and security of energy were discussed in the World Economic Forum 2009, and the report which is published from the World Energy Council 2010 stated that with improving the economics of the developing countries, the demand of water for energy improvement will be increased. So the competition between energy and water is expected to influence the water resources and its reliability [14-16]. This great development is resulted to a great pressure on the water supply in the world, so the countries try to make a control between water demands and water availability to use it for supporting the development of economy and human health in the next years. The impact of water will be so important factor in sustainable energy development in the world [17,18].
Figure 1: Percentage of water usage in different aspects of life with a maximum corresponding to power generation and irrigation [10].
In the developed countries all the effort and plans are concentrated on developing the security of energy and increasing the use of domestic energy supplies. So the suggested solutions include more depending on the electric power for transportation because generating electricity won’t depend a lot on the fuel resources. Many nations are trying to find and use alternative resources for transportation fuels such as oil sands, hydrogen, oil shale and development of natural gas supplies from gas shales [19-21]. Because of the worries about the climate change have increased , the demands of decreasing carbon dioxide and many other emission of greenhouse gas also increased, but these demands and options needs a huge using of water, so the competition for the limited fresh water resources in many regions will be increased [22,23]. A 1000-MW steam PP needs 40,000 l/s of water (3.5 billion liters/d) to keep temperature difference 8°C= 50% water usage for a big city e.g. NY. A corresponding nuclear PP will need 2.5 times this quantity ~ 9 billion l/d, due to less resulting steam temperature (Tst) and thermal efficiency (ɳth) vs. fossil plant [24,25].
Furthermore, if some power plants decrease the using of water, it may backfire. So, the environmental agencies can modulate the regulations of clean water addressing the withdrawal of cooling water for industries and especially power plants. That’s to decrease the ecological effects of thermal pollution. The changes may make many power plants to go toward closed-loop cooling towers instead of use once-through cooling. 1-2 % of PP output for fan drives. It loses 3% of water by evaporation. The cost of dry cooling tower is 4 times as expensive /kW as that of wet tower [26-28]. Even though moving to closed loop cooling aim to decrease the ecological worries of cooling systems, but this move will increase the consumption of water to the double in the power plant. Figure 2 shows the environmental effects of cooling systems [29-31]. There are other using for water in power plants such as using it for coal scrubbing and for air emission issues but using water for cooling equals 95% of total water using in the power plants. The limitations on water resources are increased in many nations, and because of climate change they are affected by demands for agriculture, domestic uses, and reductions in surface water flows [32].
The increasing in using water for agriculture specially for growing crops because of the increasing of the populations and also to cover the changes in diet which is tend to use more meat in the developing countries is evaluated to be 20-40%. Because of increasing the growth of the economy of developing countries and increasing the populations, the demands of domestic water supplies will also be increased [33-36]. The surface water flows in the midlatitude areas of the globe (the southern United States, southern Europe, northern Africa, the Mideast, India, China, Argentina, southern Africa, southeast Asia, Australia, and New-Zealand) will be reduced by around 25% because of the climate change [37]. So, these contain a lot of main places in the world that experiencing the highest economic and population growth. Using water for energy field is estimated to increase by 30-100% around the world. So, because it’s expected that the water supply will decrease by 25% in many places in the world, so new needs for water will be increased also. In U.S more that 50% of present daily water consumption and around 25% of the consumption of all current daily non-agricultural fresh water are for uses related to energy. So, it’s concluded that as the economy and populations increase, the needs and demands of energy and water will also increase [38-39].
To meet the increasing of the electrical power generation and the transportations of fuels generation, the needs of water will depend on many factors such as , the kind and number of the power plants were built, the cooling technologies that used, the kind and amount of alternative fuels that used and the required emissions of air and carbon [40]. It is expected that using water by the energy field will increase by 75% by 2035. Consumption is expected to increase, which will make the energy field the highest non-agricultural water consumption field [41]. This expected increasing in water demand for energy production and improvement over the next 20 years will happen at a time when the fresh water supplies in the world are becoming increasingly stressed. The present tendencies mention to that the requirements on the supplies in the world are increasing. especially, the capacity for storing surface water is limited and groundwater is being depleted.
The water and energy are connected. The water industry is consuming the energy intensively, and consuming electricity for desalination, pumping, and treatment of wastewater. For example, Energy calculations are estimated by 27% of all consumed water in the United States without agricultural sector [42]. Water is used to derive resource such as gas, coal, biomass etc. Energy conversion (refining and processing), transportation and it’s use for power generation. One of the problems which affects the choice of technology, sites and types of energy facilities is water availability.
Water is very important for oil production, especially in extraction and refining stages. even though, water resources are constrained very much in many regions so the competition for water is intense [43]. Water supplies have reduced because of the dryness, particularly in U.S., and are expected to increase with climate change [44]. Now the increasing demand of water for oil production will compete with water demand for energy production [45-47]. Water consumption to produce oil depends on geography, geology and recovery technique. Water is used in oil production to enhance oil recovery (EOR). Oil generation also produce a large quantity of water, on ratio near seven times the volume of produced oil. We can use the generated water for re-injection as part of EOR activities after the treatment. So, the used water is total water injected minus produced water used for injection [48]. The production of conventional oil is done by using primary, secondary, or tertiary recovery techniques [49]. We express the water use intensity (WUI) as water use per unit of produced energy, and it’s different that the recovery technology. Primary recovery can be expressed as the minimum intensity of water with an expected water use per unit of recovered oil [50]. The WUI of secondary recovery varies by location and technology [51-52] and Tertiary WUI is also highly variable with a wide range of WORs (water to oil ratio).
a) Primary/Secondary Recovery
The production of primary and secondary reaches 0.2 percent and 79.7 percent of overall production of U.S. and the waterintensity range is 1.4 gal/MMBtu for the primary and 62 gal/ MMBtu for the secondary.
b) Tertiary Using Common EOR Techniques. The range of the most common (20%) EOR techniques goes between 39 gal/MMBtu (steam injection) and 94 gal/MMBtu (CO2 injection).
Water is used in many aspects of well installation and operation; it is a primary constituent of drilling mud and the cement used to case and seal the well from non-target rock formations. Unconventional oil production using hydraulic fracturing (HF) with horizontal wells in shales has increased very much specially in U.S. So the onshore crude oil production in 2008 was almost 12% then increased to 35% in 2012 and expected to reach 50% in 2019 [53]. But using HF consumes a large volume of water under high pressure to produce oil or gas, and horizontal wells to increase contact area [54]. Water depots is the used water for hydraulic fracturing and it’s sourced from public or private water distribution sites [55,56]. Usually the source of water depots is the water from groundwater reserves or from surface water.
The Energy Information Administration (EIA) reported that natural gas especially from the formations of shale will be the main operator of development in domestic natural gas production through 2035, and it is expected that shale gas grows from 23% of supply in 2010 to 49% in 2035, more than offsetting declining production from conventional sources [57]. The consuming of water in the natural gas wells happens in small amounts during the drilling period and for drilling lubrication and cooling. So, from the production well the energy content will be recovered, according to that the total water density is effectively close to zero [58]. Directional drilling and high-volume chemically amended hydraulic fracturing techniques have enabled economic shale gas extraction; however, these processes use large quantities of water [59-61]. Also, wells produce large volumes of wastewater that requires treatment and disposal, including drilling wastewater, flow back, and produced water. In spite that the direct water consumption for operations of drilling and fracturing at the wells location but also the indirect consumption of water for supplying chain production of each well life cycle stage includes a lot of water intensive industrial sectors [62]. The indirect supply chain water use and linked environmental influences are generated across industrial actions and across watershed and state boundaries [63]. The growth of unconventional gas has made the water the first in the global discussion about shale gas. There is multistage hydraulic fracturing of the wells is required to develop the shale and tight gas tanks, and this process requires a million of water gallons for well.
Water Density:
a) The consumed water during the development (preproduction) period, and it’s primarily for hydraulic fracturing and, to a lower extent, drilling.
b) expected final recovery of natural gas from the well.
c) These factors vary by well, depending on the geology and the development decisions by the operator [64].
a) geological (maturity of shale, formation thickness).
b) Technological (horizontal vs. vertical wells, water recycling).
c) Operational (proximity of fresh-water source) [65].
The large quantities of fresh water are prerequisite to produce energy resources. With the expansion and the change of the energy sector, the mix of technologies assigned to produce fuel and electricity became able to determine the related burden of the regional water resources [66]. Renewable energy sources have very different water consumption problems. Wind and solar PV don’t use water but concentrating solar power (CSP) uses steam turbines so it has water consumption patterns comparable to or higher than conventional power plants. The geothermal and hydropower are both very large users of water [67].
a. Generating units were assigned to categories based on the technology used to generate electricity. These generationtype categories are combustion steam, combined-cycle, nuclear, geothermal, and solar thermal.
b. Cooling systems were separately categorized as either wet cooling towers or surface-water cooling systems, and the surfacewater cooling systems were subcategorized as cooling ponds, lakes, and rivers [68].
c. The rate of the water’s consumption at the thermoelectric power plants represents a small part of the water consumption in the world, but it represents a big part of the total water consumption in USA. Nevertheless, thermoelectric water consumption is expected to increase with the increasing demand of energy although the currently available data for thermoelectric water consumption are still incomplete, and the coefficients deployed to estimate consumption are contradictory.
Represents the evaporated water or the water incorporated into secondary products as a result of producing electricity from heat. Most of the water consumption at most of the thermoelectric plants are represented by the evaporation from the cooling system [69]. For instance, thermoelectric consumption has been estimated to be about 2% of the total water consumption in USA; therefore, we could infer that the usage of water in the thermoelectric plants is very important as these plants cannot operate without the consumption of water, and closing of the power plants may impose high costs on the society [70].
Extracted water is any water that is present in a reservoir with the hydrocarbon resource and is produced to the surface with the crude oil or natural gas. Extracted water is the most significant waste stream generating in the oil and gas industries, and it represents a mixture of different organic and inorganic combinations. The outcome and the influence of discharging produced water on the environment has recently become a pivotal issue of environmental concern due to the constantly increasing volume of waste all around the world in the current decade [71]. The physical and chemical properties of extracted water are different according to the geographic location of the field, the geological formation with which the extracted water has been in contact for thousands of years, and the type of hydrocarbon product being produced. The properties and volume of the extracted water can even vary through the lifetime of a reservoir. If water flooding operations are conducted, these properties and volumes may vary even more dramatically as additional water is injected into the formation [72]. About 107 million barrels of water were produced daily in offshore processes all over the world side by side with the 120 million barrels of equivalent oil in 2005. ِAlso, around forty percent of the daily water production which equals 44 million barrels was discharged offshore. As the expected oil production remains constant, the water which is being produced from such productions continues to increase. Figure 3 displays a major issue related to the oil and gas industry [73].
In oil production, water is extracted along with oil and grease and results in a produced water intensity (PWI) relative to energy production, or “water cut” which is the produced water relative to total fluids (oil and water) [75]. The volume of extracted water generated by oil and gas well can be different significantly because of many factors; such as, reservoir geology, field depth and age, well age, and recovery technology used [76-79]. The water extracted from the oil’s productions may consist of groundwater or seawater which is generally called the “source” water that is being injected to maintain the reservoir’s pressure, as well as the miscellaneous solids and bacteria. Most of the water produced from oil productions is more saline than the seawater [80]. The produced water could also include chemical additives that are used in processes of producing and drilling and in the processes of oil/ water’s separation. Treatment of chemicals are typically complex mixtures of various molecular compounds. These mixtures can include:
a) Corrosion inhibitors and oxygen scavengers to reduce equipment corrosion.
b) Scale inhibitors to limit mineral scale deposits; biocides to mitigate bacterial fouling.
c) Emulsion breakers and clarifiers to break water-inoil emulsions and reverse breakers to break oil-in-water emulsions.
d) Coagulants, flocculants, and clarifiers to remove solids [81].
The global production of extracted water is estimated to be around 250 million barrels per day comparing with around 80 million barrels per day of oil. In consequence, the water to oil ratio is around 3:1 which means that the rate of water cut is 70%. The global water cut has increased since more than a decade and it will increase constantly. Extracted water is driven up by maturing of old fields but driven down by better management methods and the introduction of new oil fields. Figure 4 shows the estimation of onshore and offshore water production since 1990, and the forecast of that in 2015 [82,83].
There are different factors that can affect the amount of produced water production on the life of a well [84]:
a) Method of well drilling.
b) Location of well within homogeneous or heterogeneous reservoirs.
c) Different types of completion.
d) Single zone and combined.
e) Type of water separation technologies.
f) Water injection or water flooding for enhancing oil recovery g) Poor mechanical integrity.
h) Underground communications.
In the fast developing shale gas industry, managing extracted water is a major challenge for maintaining the profitability of shale gas extraction while protecting public health and the environment [85,86]. The quick development of shale gas in the world has been controversial. Proponents advocate for shale gas production as an economic boom and a potential bridge to a lowcarbon future. Opponents contend that the potential for drinking water contamination from shale gas wells, negative environmental impacts of produced water management, and potential for leakage of methane, a potent greenhouse gas, outweigh any benefits [87- 89]. The technological advances in shale gas produced water, similar to the advances in hydraulic fracturing which facilitated the development of the shale gas industry, must be developed to promote expanded reuse opportunities that require higher quality water [90].
The produced water is separated from gas in the producing process, and in addition to the formation water, this water which is produced from gas includes condensed water. The water produced from gas productions contains larger amounts of low molecularweight aromatic hydrocarbons; such as, Benzene, Toluene, Ethyl benzene, and Xylene than the water produced from oil processes; therefore, the water produced from gas is more toxic than the water produced from oil productions. The studies indicate that the produced water which is discharged from gas/condensate platforms is about 10 times more toxic than the produced water that is discharged from oil platforms [91-92].
The produced water from shale gas consists of:
a) Water formation: formation water is extracted over the lifetime of the well.
b) Flow-back from hydraulic fracturing: flow-back returns to the surface during the initial weeks of well pumping after hydraulic fracturing has occurred [93].
Figure 5 shows the classifications of shale gas and the components of shale gas produced water. The quantity and quality of extracted water from shale gas wells different depending on:
a) shale gas play.
b) well location within a shale play.
c) Lifetime of the well.
The highest rate of flow-back occurs immediately after well pumping begins and diminishes over time [94].
Figure 5: Classifications of shale gas as one type of unconventional natural gas resource and indicating the two components of shale gas produced water: flow-back and formation water.
The renewable energy sources can be considered as providers whose availability changes depending on fixed or variable periods of time and also depending on the amount of energy that is not always constant. The geographical areas lacking drinking water are expected to provide great proportions of renewable energy sources, i.e., wind, solar, etc. Therefore, it is convenient to combine these renewable sources of energy with a desalination plant in order to be able to produce and supply the necessary drinking water [95-96]. Freshwater is one of a renewable resources, but while the increasing of population growth and population density has limited the ability of many local supplies to keep water quantity requirements at suitable levels of water quality. While the United Nations predictions that 2-7 billion people will face water crisis by the middle of the century so the water industry has become increasingly depending on desalination of ocean and brackish water supplies [97-98]. All of the energy cost, operational and maintenance cost, and the capital investment are considered the major contributors to the cost of water production in any of these processes. The energy cost alone is responsible for around 50% of the produced water cost [99].
The desalination technologies improve the water’s quality and they greatly reduce the problems of water shortage, and it also improve the quality of life and the economic status. Desalination technologies are classified as thermal (phase change) and membrane desalination. The major thermal distillation technologies include the Multi-Stage Flash (MSF), Multi-Effect Distillation (MED), Vapor Compression (VC), whereas the main membrane technologies are Reverse Osmosis (RO) and Electro-Dialysis (ED) and (EDR) [100]. Water desalination has become a perfect solution technologically and economically to solve the challenges associated with increasing water shortages existing in many regions of the world [101].
Multi-Stage Flash
The multi-stage flash (MSF) distillation process depends on the principle of flash evaporation, and in this process, the seawater is evaporated by reducing the pressure rather than raising the temperature. The regenerative heating, through which the seawater that is flashing in each flash chamber or each stage gives up some of its heat to the seawater that is going through the flashing process, is considered from the major methods by which the economies of the MSF technology are achieved [102]. MSF distillation is an energyintensive process which requires both thermal and electrical energy. The thermal energy is in the form of low-pressure bleed steam (1 to 3 bars) for the feed-brine heating, and medium-pressure steam for the ejectors to generate the desired vacuum in different sections of the unit. The electrical energy is needed for the driving of the unit’s various pumps; such as, recycling, cooling water, distillate product, brine blow down, condensate, and chemical dosing pumps [103].
In this kind of process, the feed seawater moves in heat exchangers through the stages and gains some heat that helps to decrease the external thermal energy needed for hot brine and also to condense the water vapor for collection as fresh water in each stage then external heating source is supplied to the intake preheated seawater to raise its temperature to the required top brine temperature of 90.1 to 11oC [104]. After that the heated brine water will be moved through stages that are held at lower pressure in which a small amount of water flashes to vapor in each stage and the remaining brine flows to the next stage for further flashing until it is finally discharged. The vapor from each stage is condensed and collected as fresh water [105]. Figure 6 shows Schematic diagram of MSF unit.
The multiple-effect distillation (MED) process is the oldest desalination method [9] and is very efficient thermodynamically. This process consists of a series of stages that are maintained at decreasing levels of pressure [106] Figure 7.
Distillation plants that used vapor compression depend on the heat generated by the compression of water vapor to evaporate salt water, and there are two methods (1) mechanical vapor compression (MVC), (2) thermo vapor compression (TVC). The feed water enters the VC process by a heat exchanger, and vapor will be generated in the evaporator and compresses by mechanical (MVC) or thermal (TVC) means. Compression the vapor raises its temperature by a sufficient amount to serve as the heat source. Then the concentrated brine will be removed from the evaporator vessel. This flow is then split, and a portion is mixed with the incoming feed and the remainder is pumped to the waste [107]. Figure 8 shows a schematic diagram of VC (MVC and TVC) units.
Reverse Osmosis
Reverse osmosis (RO) process, the osmotic pressure is overawed by put on external pressure higher than the osmotic pressure on the seawater. Therefore, water flows in the reverse direction to the natural flow across the membrane, leaving the dissolved salts behind with an increase in salt concentration. No heating or phase separation change is necessary [108-111]. Figure 9 is a diagram of a RO system. RO is a developed technology that is commonly used desalination technique [112-116].
The present installed desalination capability using renewable energy is negligible comparing with the world’s capacity. As mentioned in many studies the aim of the usage of solar energy (SE) is a 3–5% share of new installations in the world desalination market by 2016. In the world, many small-scale RE-driven desalination plants were installed and most of them were successfully worked and showed that they need very simple maintenance [117- 119]. Solar energy and geothermal energy (GE) will be a stabile alternative source because it is everlasting, abundantly available and is environmentally friendly [120-121]. CSP is technology that generate electricity which uses heat provided by solar irradiation concentrated on a small area. Using mirrors, sunlight is reflected to a receiver where heat is collected by a thermal energy carrier Figure 10.
Figure 10: Available CSP Technologies: (1) Solar power tower; (2) Parabolic trough collector (3) Linear Fresnel reflector (4) Parabolic dish systems [122].
Identify and quantify emerging energy and water challenges and interdependencies, then identify the relevant science and technology needed.
a) Advanced cooling technologies.
b) Water reuse and recovery.
c) Use of nontraditional sources of process and cooling water [123].
Challenge Related to Cooling Requirements of Thermal Power Generation are:
a) The amount of freshwater taken into cooling system.
b) Volume and salinity of water that is eventually discharged [124].
Short falls in water supplies could result in restrictions or limitations on both thermal and hydroelectric capacity. In periods of serious drought, the ability of major thermal and nuclear PP could be threatened. Natural gas and heavy oil recovery are fairly bag water users. They should focus on recovering and reusing those waters, to minimize the fresh water used.
Improving dry, hybrid, and other alternative cooling technologies and carbon sequestration approaches would lower future water consumption and reduce the water footprint of power plants. Addressing issues- such as electric grid integration, cost, and dispatch ability-that are limiting the implementation of lowwater-use, renewable energy technologies, could reduce both water consumption and carbon emissions [125]. Major scale-up of alternative transportation fuels must consider approaches that use less fresh water than current methods, and must improve water use efficiency in growing, mining, processing and refining future fuel resources [126]. With the increase in energy demand and the expected shortage of the fossil fuel with time the need for sustainable resources increases. Hence, this is initially handled by using clean fuels, utilization of waste heat [127] and adopting different configurations [128], where resources and environment are conserved.
Extracted water is challenging to treat due to its complex physicochemical composition, which may vary over the lifetime of the shale gas well. Treatment technologies should be designed for these potential changes in produced water quality over time. Constituents of concern in produced water[129] include dissolved and suspended organics, measured as total oil and grease; suspended solids, such as formation solids, corrosion and scale products, and bacteria; production chemicals, which may contain friction reducers, biocides, and corrosion inhibitors from the hydraulic fracturing fluid; [130] naturally occurring radioactive material, specifically barium and radium isotopes; and total dissolved solids (TDS), including hardness and heavy metals. Reuse of wastewater and use of non-traditional water, including seawater, brackish ground water, and produced water, could be increased to meet water demands in many sectors. New water treatment technologies will be needed that can meet the water quality requirements at much lower energy use. Improvements in materials that minimize fouling would reduce the need for higher-quality waters, and significantly expanding opportunities to replace fresh water with lower-quality waters [131,132]. Generally, Treatment technologies available to treat HF fluids are grouped as basic pre- and post-treatment or as advanced treatment technologies. The pre-/post-treatment category includes physical separation (filtration, sedimentation), coagulation-flocculation, and some disinfection processes (using chlorine dioxide, ozone, or ultraviolet light) [133].
A. Engineers and scientists have the role to develop more efficient approaches to redesign ,use and reuse water in ways that will reduce the footprint of energy and to support continued economic development and to maintain the sustainability of energy, water, and all other natural resources.
B. Energy and water are two needs having a common cause: New technologies to assure a sustainable future.
C. The water and energy are linked. The water industry is energy-intensive, consuming electricity for desalination, pumping, and treatment of wastewater.
D. Water is very important for oil production, especially in extraction and refining stages. Even though, water resources are constrained very much in many regions so the competition for water is intense.
E. Renewable energy sources have very different water consumption problems. Wind and solar PV don’t use water, but concentrating solar power (CSP) uses steam turbines so it has water consumption patterns comparable to or higher than conventional power plants. The geothermal and hydropower are both very large users of water
F. The volume of extracted water generated by oil and gas well can be different significantly because of many factors, such as, reservoir geology, field depth and age, well age, and recovery technology used.
G. In the fast developing shale gas industry, managing extracted water is a major challenge for maintaining the profitability of shale gas extraction while protecting public health and the environment.
H. Energy cost, operational and maintenance cost, and capital investment are the main contributors to the water production cost of any of these processes. The energy cost is responsible for about 50% of the produced water cost.
I. Desalination technologies improve water quality, greatly reduce water shortage problems, and improve quality of life and economic status.
Bio chemistry
University of Texas Medical Branch, USADepartment of Criminal Justice
Liberty University, USADepartment of Psychiatry
University of Kentucky, USADepartment of Medicine
Gally International Biomedical Research & Consulting LLC, USADepartment of Urbanisation and Agricultural
Montreal university, USAOral & Maxillofacial Pathology
New York University, USAGastroenterology and Hepatology
University of Alabama, UKDepartment of Medicine
Universities of Bradford, UKOncology
Circulogene Theranostics, EnglandRadiation Chemistry
National University of Mexico, USAAnalytical Chemistry
Wentworth Institute of Technology, USAMinimally Invasive Surgery
Mercer University school of Medicine, USAPediatric Dentistry
University of Athens , GreeceThe annual scholar awards from Lupine Publishers honor a selected number Read More...