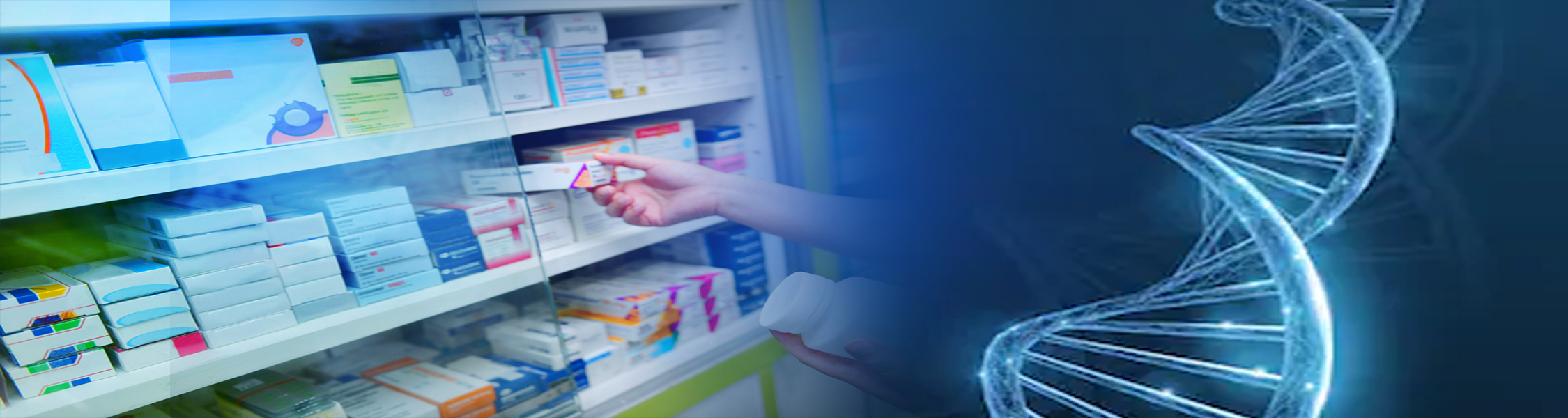
ISSN: 2690-5760
Inass A Sadawe1, Abdulhakim M Qabaj2, Nisreen H Meiqal1, Salah M Bensaber1, Abdulhamid R Hamid3, Massaud Salem Maamar4, Anton Hermann5 and Abdul M Gbaj1*
Received: December 21, 2020 Published: January 11, 2021
Corresponding author: Abdul M Gbaj, Professor of Genetics and Biochemistry, Department of Medicinal Chemistry, Faculty of Pharmacy, University of Tripoli, Libya
DOI: 10.32474/JCCM.2021.02.000147
Thymus capitatus extract has been considered a promise as antiviral agent against COVID‑19 viruses. We hypothesized that Thymus capitatus components may interact with key protein targets of COVID‑19 (coronavirus 2 (SARS-CoV-2) causing severe acute respiratory syndromes. Molecular docking analysis was carried out using 31 components of Thymus capitatus with SARS-CoV-2 protease enzyme (6LU7) and spike glycoprotein (6VSB). The compounds with the best normalized docking scores to protease enzyme were allo-Aromadendrene (-6.3 kcal/mole), spathulenol (-6.6 kcal/mole) and ledene (-6.8 kcal/mole). The best docking ligands for spike glycoprotein were allo-Aromadendrene (-6.6 kcal/mole), spathulenol (-6.6 kcal/mole) and ledene (-7.3 kcal/mole). All Thymus capitatus components may act synergistically to produce the therapeutic action. Thymus capitatus components may potentiate the effect of prednisolone, azithromycin and other medicines used to treat COVID-19 patients.
Keywords: COVID-19; Corona Virus; Molecular Docking; Antiviral; Thymus Capitatus
The outbreak of Coronavirus SARS-Cov-2 disease (COVID-19) in Wuhan (China) in December 2019 and its worldwide spread has led in some cases to heavy disease symptoms finally causing deaths [1,2]. During the year 2020, the disease has affected almost all countries on the globe. The majority of people infected with the COVID-19 virus face mild medium respiratory symptoms and recover mostly without any treatment [3,4]. Elderly people and people with chronic medical conditions such as diabetes, chronic respiratory disease, cardiovascular disease, or cancer are expected to develop more serious symptoms [5, 6]. Young people and adults who have no serious medical conditions are requested to self-manage their symptoms in isolation possibly taking a pain killer such as paracetamol or using hyperthermia and lifestyle modifications such as increased rest and taking good supply of water [7,8]. However, most of the presen [9, 10]. COVID-19 symptoms usually start with dry cough, fever, and dyspnea, which is similar to other viral respiratory diseases such as ordinary flu. Consequently, the diagnosis of COVID-19 becomes difficult. Generally, the incubation period of COVID-19 is approximately a few to 15 days. SARS-Cov-2 has a dramatic transmission capability [9, 10. This could be related to the fact that a significant number of patients are asymptomatic (up to 60%) who accidentally participate to the spread of the disease [11, 12]. In COVID-19 the infection from mild upper respiratory tract problems to severe acute respiratory syndromes which may lead to respiratory failure and shock which in addition may cause multiple organ failure. Other accompanying features might arise such as fatigue, diarrhea, headache, or lymphopenia and cardiovascular symptoms [13,14].
Corticosteroids, as generally used immunosuppressants and anti-inflammatory agents play an significant role in the treatment of critical diseases [15, 16]. Previous studies showed that corticosteroid was used to treat coronavirus diseases, including Middle East respiratory syndrome (MERS) and severe acute respiratory syndrome (SARS) [17]. Studies have demonstrated that cytokine reactions caused by the enormous release of cytokines were major cause of death in a number of cases of SARS and MER [18, 19]and corticosteroids could have a positive antagonistic effect on the cytokine storm [20]. Azithromycin has immunomodulatory and antiviral properties that might be of attention in the treatment of COVID-19. The use of this macrolide has been connected with the progression of clinical therapy in other viral infections and community acquired pneumonia [21].
Azithromycin has been shown to exert in vitro activity against SARS-CoV-2 and may operate at many points of the viral cycle. Additionally, the immunomodulation of Azithromycin improved clinical outcomes by its capability to preserve epithelial integrity, down regulate cytokine production and avoid lung fibrosis and hence could play a role in the hyperinflammatory stage during COVID-19 infection [2, 23].
Current confirmation showed that nutritional supplementation might have a supportive role in COVID-19 patients. Administration of higher than recommended daily doses of nutrients such as vitamins C, D, E, omega-3 fatty acids and Zinc exert a valuable effect by significantly reducing SARS-CoV-2 viral load and the time of hospitalization [24, 25]. These nutrients are known for their immunomodulatory and antioxidant ability. Deficiencies of such nutrients could lead to immune dysfunction, and enhance vulnerability to pathological infection. Dietary deficiency of vitamins and minerals has been found in high-risk groups of COVID-19 patients, such as the elderly by increasing morbidity and risk of mortality [26]. Natural products and herbal medicine are employed to treat respiratory infection and several have been approved as drugs, over-the-counter or food additives. The usual low to negligible toxicity makes herbal medicines and natural product reasonable prophylactic candidates for treatment. Using in silico technology to study natural products has been found utterly effective to find blockers of enzyme function and membrane receptors of human coronavirus [27, 28]. The symptoms of COVID-19 are characteristic exhibiting uncontrolled inflammation, such cytokine release syndrome, and hence anti-inflammatory herbs are able to suppress such often deadly symptoms. Digestive enzymes, low pH in the gastric vicinity or the gut microbiome have less effect on the bioavailability of natural products and herbs in comparison to antibody and other prophylactics [29, 30].
Thyme is a plant with a long history in traditional medicine in Libya that has antiseptic, antimicrobial, and antiviral properties. It also has useful effects on the cardiovascular and respiratory system [31-33]. Thymus capitatus belongs to the thymus genus and the Lamiaceae family. This plant is small, perennial and grows in hot and dry climates like Libya, Tunisia, Algeria etc [34, 35]. Thymus capitatus contains bioactive compounds such as phenols, phenolic acids, terpenes and flavonoids [36-38]. Thymus capitatus is containing a rich source of vitamins, particularly vitamin C, vitamin A, and vitamin B6. Furthermore, in the leaves of this plant, minerals such as calcium, iron, potassium, manganese, magnesium, and selenium are found in abundance [39].
Molecular modeling is a recognized computational tool to aid early drug discovery and development. It is used to generate ideas of a compounds or macromolecules 3D conformation, protein–ligand interactions, and allows forecasts about biological activities. The integration of molecular modeling in drug or vaccine design can help in early drug or vaccine discoveries [40-42].
The main aim of this study was to investigate the effects of Thymus Capitatus extract on COVID‑19 patients clinically to provide information if it can be used as a clinically compatible option for adjuvant therapy. We also performed in-silico studies on two targets -protease enzyme and spike glycoprotein - to collect information if they are suited as targets by computational drug repurposing procedures to allocate appropriate inhibitory agents.
The study was performed between October 1st and November 30th, 2020, a total of 60 cases were investigated at Arada polyclinic, Tripoli Libya. All the patients were informed and consented. Sixty COVID-19 patients were enrolled in the study. The age group of patients was from 40-85 years. The patients were treated at the Out Patient Department (OPD) of Arada polyclinic, Arada, Tripoli Libya.
All patients were screened for COVID-19 symptoms by polymerase-chain reaction (PCR) as well IgM and IgG antibody tests within 5 days of the study. Additionally, a history of diabetes, chronic obstructive pulmonary disease COPD, coronary artery disease CAD, and use of immunosuppressants were recorded for risk evaluation. All patients came to the clinic with pulmonary infiltrate bilateral atypical pneumonia (walking pneumonia). Patients diagnosed with clinical symptoms showed: sore throat (pharyngitis), feelt tired (fatigue), had chest pain, mild to severe chills, high-grade fever, persistent cough that can be dry or produce colored mucus, sneezing dyspnea and severe headache. In addition, high-resolution computed tomography HRCT, also called thin-section scan (CT scanning) was used to confirm pneumonia diagnosis.
The patients were divided into two groups: the first group consisted of 60 patients (45 males and 15 females) to whom Thymus capitatus (10 g/200 ml boiled water containing the 10g of stem and leaves of Thymus capitatus) was administered. In addition, patients were given prednisolon 40 mg daily for 7 days and then the dose gradually decreased by 10 mg every 7 days until the last dosage of 10 mg for 7 days. Azitromycine 500 mg tablets were used once a day for 6 days. Ceftriaxon (Rocephin 1g powder for injection solution) was given intramuscular twice a day for a week. Ipratropium (250 μg/1ml) and salbutamol (5mg/2.5ml) were inhaled using a nebulizer every 8 hours for two weeks. The patients were also treated with standard prophylactic LMWH Nadroparin calcium (Fraxiparine injection®) subcutaneously as a single daily dose of 0.3 ml (2,850 IU) or 0.6 ml (5700IU/0.6mL) for at least 7 days dosage. In addition, one tablet daily of Vitamin C (Ascorbic Acid) 500 mg, Vitamin D (from Cholecalciferol) 10 μg and zinc 15 mg were supplied for two months.
The starting geometry of Thymus capitatus constituents was downloaded from Pubchem (https://pubchem.ncbi.nlm.nih.gov) into Chem3D Ultra software (version 8.0, Cambridge soft Com., USA). The optimized geometry of Thymus capitatus constituents with the lowest energy was used for molecular dockings. The crystal structure of COVID-19 main protease in complex with an inhibitor N3 (6LU7) was downloaded from the Protein Data Bank http://www.rcsb.org/structure/6LU7 and prefusion 2019-nCoV spike glycoprotein with a single receptor-binding domain (6VSB) was downloaded from the Protein Data Bank http://www.rcsb. org/structure/6VSB. Molecular dockings of Thymus capitatus constituents with 6LU7 and 6VSB were accomplished by Auto Dock 4.2 software from Scripps Research Institute (TSRI) (http:// autodock.scripps.edu/). Firstly, polar hydrogen atoms were added into protein molecules. Then, partial atomic charges were calculated using Kollman methods for protease enzymes and spike glycoprotein with Thymus capitatus constituents [43] . In the process of molecular docking, the grid maps of dimensions: (60Å X 60Å X 60Å) and (36.8Å X 64.6Å X 60Å) for 6LU7 and 6VSB, respectively, with a grid-point spacing of 0.376Å and the grid boxes centered were used [44]. The number of genetic algorithm runs and the number of evaluations were set to 100. All other parameters were default settings. Cluster analysis was performed on basis of docking results by using a root mean square (RMS) tolerance of 2.0Å, dependent on the binding free energy. Lastly, the dominating configuration of the binding complex of Thymus capitatus constituents with protease enzyme and spike glycoprotein fragments with minimum binging energy were determined which relied strongly on the information of 3D-structures of the protease enzyme and spike glycoprotein binding site and ultimately generated a series of protease enzymebinding complexes and spike glycoprotein-binding complexes.
Patients who had recovered from COVID-19, 95.0% reported persistence of at least 1 symptom, particularly very mild dyspnea and mild fatigue. 5% (3 patients) died a 84 years old man with diabetes and hypertension, a lady 72 years old with myopathy and a 45 years man. Limitations of the study include the lack of information on symptom history before acute COVID-19 illness and the lack of details on symptom severity. Furthermore, this is a single-center study with a relatively small number of patients (60) and without a control group of patients whom did not use Thymus capitatus.
Thymus capitatus contains a variety of functional chemical groups including phenols, phenolic acids, terpenes and flavonoids. The most important compounds in Thymus capitatus include 4-thujanol, allo-Aromadendrene, α-copaene, α-pinene, α-terpineol, β-bisabolene, bronyl, β-phellandrene, β-pinene, carvacrol, carvenone, eugenol, cis-carveol, β-cis-ocimene, limonene, geraniol, neral, spathulenol, linalool, p-cymene, thymol, terpiene-4-ol, terpinolene, p-cymen-8-ol, trans-piperitol, carvotanacetone, cis-dihydrocarvone, champhene, α-humulene, 3-octanol and ledene (viridiflorene). For example, thymol exhibits numerous biological effects such as antioxidant, anti-inflammatory, free radical scavenging properties and immunomodulating properties. Also, carvacrol exhibits antimicrobial, antioxidant activities and anticarcinogenic effects [45]. Furthermore, many studies have confirmed the effect of thymol and carvacrol decreasing the proinflammatory cytokines: Interleukin (IL)-1β, IL-6, and tumor necrosis factor (TNF)-α [46, 47].
It is reported that in SARS-CoV-2 infection, the level of AngII increases [48]. The binding of AngII to AngII type 1 receptors (AT1R) is a vital mediator for majority of pro-inflammatory effects. The AngII type 2 receptor (AT2R) is activated by AngII which activates some inflammatory cells. AT1R activation induces the overexpression of chemokines, adhesion molecules, and cytokines via special pathways such as NF-kB up-regulation. NF-kB plays an essential role in the regulation of numerous pro-inflammatory genes [49, 50]. It has been reported that herbal thyme acts as a negative regulator of NF-kB. Furthermore, other studies showed that the plasma levels of most pro-inflammatory cytokines, such as TNF, IL1B, IL7, IL1RA, IL8, IL9, IL10, basic fibroblast growth factor (FGF), and other pro-inflammatory factors were increased in COVID-19. Consequently, thyme fits as an inhibitory agent of pro-inflammatory cytokines [51, 52]. Hence, Thymus capitatus can be considered as adjuvant therapy for COVID-19 as described in the literature [53, 54].
Thymus capitatus contains many phenolic compounds such as thymol and carvacrol which were used traditionally in Libya to treat chronic bronchitis, sore throat, cough, asthma, colds, and other respiratory infections. Thyme is an expectorant, antiseptic, and antispasmodic herbal medicine with respiratory clearance nature [55]. During COVID-19 infection, acute lung injury and acute respiratory distress syndrome are the major reason for COVID-19 respiratory mortality. Damage of epithelial and endothelial respiratory cells, neutrophil infiltration, an extended-release of pro-inflammatory mediators, and pulmonary edema are characteristic for COVID-19 patients. Severe COVID-19 patients are characterized by a cytokine storm with increased serum levels of pro-inflammatory cytokines [56]. Cytokines such as TNF-α and IL-6 activate neutrophils and other inflammatory cells in the lung. Cytokine release leads to respiratory endothelial dysfunction which results in microvascular permeability and protein escape proceeded by hypoxemia. TNF-α and IL-6 are components of an inflammatory response and have been shown to encourage the incidence of acute lung injury and acute respiratory distress syndrome [56]. Some studies [46,47] indicate the immunomodulatory effect of thymol in lipopolysaccharide-induced acute respiratory distress syndrome through TNF-α and IL-6 suppression in the broncho-alveolar lavage fluid (BALF). NF-κB, a protein complex that triggers a pro-inflammatory signaling pathway in the lung leading to cytokine release is overexpressed in acute lung injury and acute respiratory distress syndrome [57, 58]. Studies have shown that thyme extract is a negative regulator of NF-kB p65 and NF-kB p52 transcription factors whereby pro-inflammatory cytokines (IL-1 beta and IL-8) are reduced. Further studies also established that thyme extract acts as an inhibitor of cytokines IL-1-beta and IL-8, at both the mRNA and the protein level in normal human epithelial cells [59]. Thymol can also decrease the recruitment of inflammatory cells like neutrophils into bronchoalveolar lavage fluid. Thymol inhibits NF-κB activation [59] which is a transcription regulator of inflammatory and immune response genes [59] and hence can decrease the severity of acute lung injury and acute respiratory distress syndrome. Carvacrol, another active constituent of thyme has been also shown to have a therapeutic effect on acute lung injury and acute respiratory distress syndrome. The remedial effect of carvacrol on the acute respiratory distress syndrome is through decreasing inflammatory cytokines, reducing the number of inflammatory cells in bronchoalveolar lavage fluid, and by inhibition of NF-κB [60, 61]. As a result, Thymus capitatus extract is suggested to have an ameliorating effect on respiratory symptoms of COVID-19 patients. The most common outline of coagulopathy seen in patients with COVID-19 is characterized by elevation in fibrinogen and D-dimer levels, and mild prolongation of prothrombin time/ activated partial thromboplastin time. Thymol has antiaggregant ability and it is able to inhibit platelet aggregation induced by thrombin, collagen, adenosine diphosphate and arachidonic acid [62].
In Libya some people use Thymus capitatus extract as a remedy for hypertension and other cardiovascular diseases. Thymus capitatus has effective components such as thymol and carvacrol against oxidative agents causing cardiovascular disease [63]. Cardiovascular damages contribute to the morbidity of COVID-19 patients. Patients with cardiovascular disease (CVDs) are in addition susceptible to develop severe COVID-19 symptoms, in particular acute myocardial injury [64, 65]. SARS-COV-22 appears able to provoke myocardial injury via a cytokine storm connected with a rigorous response by type 1 and type 2 T helper cells [64, 65]. Furthermore, COVID-19-induced hypoxemia [66] and respiratory collapse could lead to myocardial dysfunction [64, 65]. Cytokine storms could provoke multiple organ failure and increase inflammatory chemokines such as IL-6, IL-2, IL-7, interferon- γ, (TNF)-α. Cardiovascular injury correlates with elevated inflammatory markers [64, 65]. Moreover, the oxidative stress generated by SARS-CoV-2 might be a source of heart injures [64, 65]. Thymus capitatus contains antioxidant components, and studies have shown that an appropriate dose of antioxidants can improve heart damage caused by COVID-19 infection [67]. It has been also demonstrated that thymol and carvacrol, derivatives of Thymus capitatus, have anti-inflammatory effects [68, 69]. Taken together it appears that Thymus capitatus and its derivatives can protect cardiovascular damages induced by SARS-COV-22.
Molecular docking analysis
Table 1 shows the binding energies of Thymus capitatus constituents with SARS-CoV-2 protease enzyme (6LU7) and spike glycoprotein (6VSB) obtained by the molecular docking strategy. Molecular docking of Thymus capitatus constituents with SARS-CoV-2 protease enzyme (6LU7) and spike glycoprotein (6VSB) were performed using Auto Dock 4.2. Thymus capitatus constituents and protease enzyme (6LU7) and spike glycoprotein (6VSB). Thymus capitatus constituents and protease enzyme (6LU7) and spike glycoprotein (6VSB) were kept as flexible molecules and were docked onto seven forms of protease enzyme (6LU7) and spike glycoprotein (6VSB) to obtain the privileged binding site of Thymus capitatus constituents. The molecular docking results are shown in Table 1. The modeling studies indicate van der Waals, hydrogen bonding (Table 1) and electrostatic interactions between Thymus capitatus constituents with protease enzyme (6LU7) and spike glycoprotein (6VSB). The contribution of van der Waals and hydrogen bonding interaction is much greater than that of electrostatic interaction because the sum of van der Waals energy, hydrogen bonding energy and desolvation free energy is larger than the electrostatic energy [70, 71]. The Thymus capitatus constituents ledene and protease enzyme (6LU7) interactions are shown in Figure 1. The hydrophobic interaction of ledene with phe294 is the observed tendency of the nonpolar ability of ledene to aggregate in an aqueous solution and exclude water molecules around the protease enzyme. Ledene causes a segregation of water and nonpolar substances, which maximizes hydrogen bonding between water molecules and minimizes the area of contact between water and ledene molecules. In terms of thermodynamics, the hydrophobic effect is the free energy change of water surrounding a ledene molecule. The positive free energy change of the surrounding solvent designates hydrophobicity, while a negative free energy change implies hydrophilicity. The highest binding energies for Thymus capitatus constituents with protease enzyme (6LU7) were -6.8, -6.6 and -6.3 kcal/mol for viridifloren, spathulenol and allo-aromadendrene, respectively. The highest binding energies of Sesquiterpenes for Thymus capitatus constituents with spike glycoprotein (6VSB) were -7.3, -6.6 and -6.6 kcal/mol for viridifloren, spathulenol and allo-aromadendrene, respectively. Sesquiterpenes are lipophilic in nature and have a high log P value indicating the significance of lipophilicity for the interaction with the active site. Viridiflorene, spathulenol and allo-aromadendrene are carbotricyclic sesquiterpenes and studies have shown have shown that many carbocation intermediates in terpenoid cyclization cascades are subject to electron delocalization through bond coupling or through hyperconjugation and this directs the cyclization trajectory. Carbocation intermediates of viridiflorene are stabilized in the active site of a terpenoid cyclase by weakly polar interactions involving charge−dipole, charge−charge, and charge−quadrupole interactions with properly oriented amino acid side chains. In addition, carbocation intermediates can be stabilized by considerable partial negative charges generated by a ring π electrons of the aromatic side chains of tyrosine, phenylalanine, and tryptophan through cation-π interactions [72-74].
Figure 1: (A) Interaction model between ledene (green) with 2019-nCoV protease (6LU7) active site. Ledene interacts mainly via hydrophobic bonding. (B) Solid structure of the 2019-nCoV protease (6LU7) showing ledene in the binding cleft.
Table 1: Binding energies of Thymus capitatus constituents with protease enzyme (6LU7) and COVID-19 spike glycoprotein (6VSB) obtained from molecular dockings. The unit of all energies (ΔG) is kcal/mol.
The obtained results using computational drug repurposing offer an efficient way to find novel applications for already known drugs [75]. Molecular docking and binding free energy calculations for sesquiterpenes can be used to forecast drug-target interactions and binding affinity. The appearance of resistance to existing antiviral drugs or vaccines is a major challenge in antiviral drug development. The drug repurposing technique allows finding novel antiviral agents within a short period in order to overcome the challenges in antiviral therapy. Computational drug repurposing has previously been used to recognize drug candidates for viral infectious diseases like ZIKA, Ebola, influenza and dengue infections. These methods were also utilized to recognize possible drugs against MERS-CoV and SARS-CoV [76,77] and following the COVID-19 outbreak, computational repurposing can be used for finding COVID-19 antiviral agents. The Thymus capitatus sesquiterpenes are C15-terpenoids built from three isoprene units and are found mainly in higher plants as well as in fungi and marine organisms. Naturally, they are available as hydrocarbons or in oxygenated forms including alcohols, lactones, aldehydes, ketones and acids. Sesquiterpenes also include essential oils and aromatic constituents with several pharmacological activities. Studies report that sesquiterpenes have anti-inflammatory, anticancer, antidiabetic, antinociceptive and antimicrobial activities [78, 79]. They also effect the central nervous system that make them possible targets for the development of novel therapeutics and of usage of medical intentions [78, 79].
The results obtained by molecular modeling and by clinical use of Thymus capitatus extract are very promising also for fighting covid-19 viruses. It has been reported that sesquiterpenes directly inactivate herpes virus and might impede with virion envelope structures or mask viral structures that are essential for adsorption or entry into host cells. A virucidal activity of Melaleuca armillaris essential oil has been reported recently [80, 81] and dissolution of the herpes simplex virus envelope by treatment with oregano essential oil has been described [82]. Thus different mechanisms of antiviral activity of many essential oils and compounds of essential oils including sesquiterpenes seem to be available. It has been also reported that Santolina insularis essential oil causes inactivation of herpesviruses and prevention of cell-to-cell spread [83, 84]. Sesquiterpenes, were found to inhibit the severe acute respiratory syndrome caused by coronavirus [85, 86], cytomegalovirus [87], and rhinovirus [88]. Furthermore, it has been reported that the chemical composition Thymus capitatus essential oil has antioxidative and antimicrobial activities [68, 89]. These herbal species were also found to be contained in thymol and its isomer carvacrol, followed by their biosynthetic precursors, terpinene and p-cymene as main compounds [68, 89]. In addition, literature data indicate that the powerful bioactive potential of Thymus capitatus constituents is principally related to the high proportion of phenolic compounds such as thymol and carvacrol [90]. Anti-inflammatory, antibacterial, antifungal, cytotoxic, insecticidal and herbicidal properties of thymol and carvacrol were studied previously [90].
In addition, numerous vaccines were recently developed by aiming at the exploration of vaccines to prevent and treat 2019‐nCoV. Computational‐based technology has given the researchers the tools to explore and identify potentially effective drug and/or vaccine candidates which can efficiently shorten the time and diminish cost.
Essential oils and their components are known to be active against a wide variety of microorganisms. Many chemical constituents present in Thymus capitatus might contribute to antiviral activity. COVID-19 increasing oxidative stress, inflammations, and disturbance of the renin-angiotensin system, lead to damage, particularly the respiratory and the cardiovascular system. Thymus capitatus and its bioactive components suppress the negative effects of the renin-angiotensin system in COVID-19 patients. Moreover, many components of Thymus capitatus decrease pro-inflammatory cytokines such as IL-6 and TNF-α. Hence, it can decrease the severity of acute lung injury and the acute respiratory distress syndrome. Thymus capitatus extract reduces oxidative stress due to its antioxidant properties that can reduce adverse cardiovascular effects during COVID-19 infection. Finally, we conclude that Thymus capitatus has antioxidant, anti-inflammatory, and immunomodulating properties and diminishes side effects in COVID-19 patients as adjuvant.
The authors gratefully acknowledge the support and valuable suggestions obtained from Sir Khaled AB Diab (Judicial Expertise and Research Centre, Tripoli, Libya Tripoli, Libya).
Bio chemistry
University of Texas Medical Branch, USADepartment of Criminal Justice
Liberty University, USADepartment of Psychiatry
University of Kentucky, USADepartment of Medicine
Gally International Biomedical Research & Consulting LLC, USADepartment of Urbanisation and Agricultural
Montreal university, USAOral & Maxillofacial Pathology
New York University, USAGastroenterology and Hepatology
University of Alabama, UKDepartment of Medicine
Universities of Bradford, UKOncology
Circulogene Theranostics, EnglandRadiation Chemistry
National University of Mexico, USAAnalytical Chemistry
Wentworth Institute of Technology, USAMinimally Invasive Surgery
Mercer University school of Medicine, USAPediatric Dentistry
University of Athens , GreeceThe annual scholar awards from Lupine Publishers honor a selected number Read More...