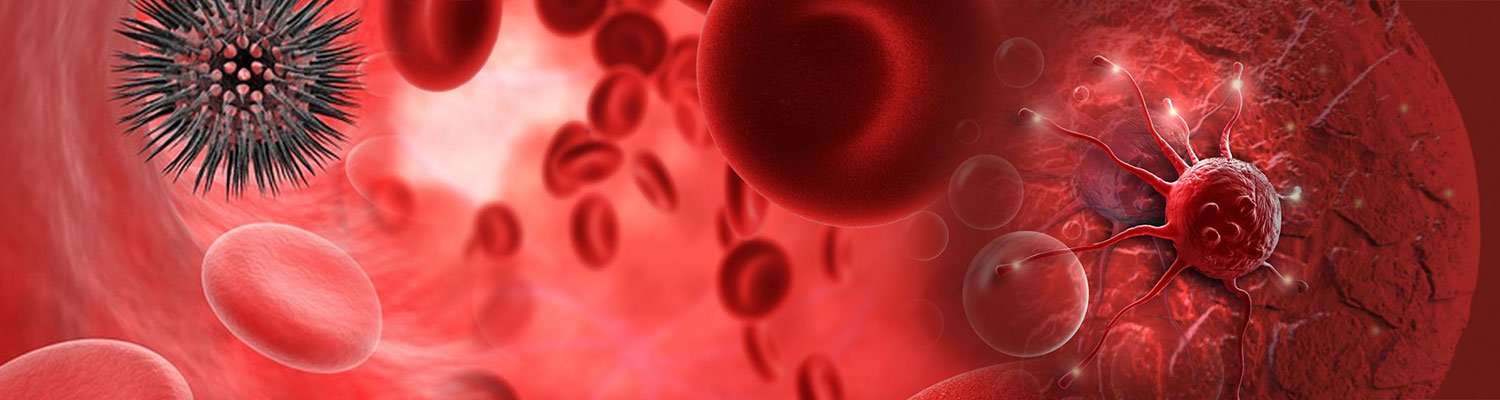
ISSN: 2638-5945
Xi Jin1#, Bandar A. Alenezi2#, Gang He3, Hongwen Ma1, Qiang Wei1, and Youqiang Ke1, 2, 3*
Received: November 25, 2021 Published: December 6, 2021
Corresponding author: Professor Youqiang Ke, Department of Molecular & Clinical Cancer Medicine, Liverpool University, United Kingdom.
DOI: 10.32474/OAJOM.2021.05.000206
The fatty acid receptor peroxisome proliferator-activated receptor gamma (PPARγ) is a transcription factor, which includes two isoforms named PPARγ1 and PPARγ2 respectively. In human body, PPARү involves in metabolic disorder, neurodegenerative disease and inflammation. Recent advance in PPARү study has led to the discoveries of several genes that are regulated by PPARγ in prostate cancer cells. Evidence showed that PPARү plays important roles in development and in malignant progression of prostate cancer. In this mini-review, we described the PPARγ structure and summarized their involvement in different diseases. Our focus is on the roles of PPARγ isoforms in prostate cancer.
Peroxisome Proliferator-Activated Receptor Gamma (PPARγ) is a transcription factor that is ligand dependent and is a member of the nuclear hormone receptor superfamily. PPARγ is expressed in two isoforms: PPARγ1 and PPARγ2, the latter contains thirty extra amino acids (Figure 1). Both synthetic and endogenous ligands can band to and activate PPARγ [1]. When activated, PPARγ is translocated into the nucleus and forms a heterodimer with the retinoid X receptor (RXR), where it serves as a transcriptional regulator of genes via DNA binding [2]. It is well established that PPARγ plays a critical role in adipocyte differentiation, the inflammatory response, and peripheral glucose consumption. PPARγ agonists are frequently utilised to treat type II diabetes [1]. Diabetes type II is the most prevalent endocrine-metabolic condition worldwide, characterised by insulin resistance and insulin secretion abnormalities. PPARγ agonists were utilised to sensitise tissues (muscle, adipose tissue, and liver) to insulin stimulation. However, these PPARγ agonist medicines were associated with significant side effects such as increased weight, oedema, heart failure, and an increased risk of myocardial infarction [3]. The role of PPARү in prostate cancer (PCa) has been controversial. Initially, it was believed that PPARγ functioned as a tumour suppressor in prostate cells since agonist ligands suppressed the proliferation of PCa cells. However, further investigations revealed that these agonists suppressed cell growth in a manner independent of PPARγ [4-8]. Furthermore, PPARγ expression rises with the grade/stage of cancer cases [9-11]. These results suggested that it is not a tumour suppressor. In the contrary, studies also find PPARγ activity may contribute to the development and the progression of the prostate cancer. While a tumour suppressor expression level frequently reduced as the develop and progress of the malignancies, PPARγ expression level appeared to be significantly increased with elevated PCa stage and grade, strongly implying that it is cancer-promoter or oncogene. For example, it was discovered by immunohistochemical staining that PPARγ expression was significantly greater and more intense in prostate cancer and prostatic intraepithelial neoplasia (PIN) tissues than in benign prostatic hyperplasia (BPH) and normal prostate tissues samples [11]. Similarly, utilising more tissue samples in a separate investigation by a different group, it was discovered that PPARγ expression was substantially higher in advanced PCa tissues than in low-risk PCa and BPH specimens (P <0.001) [10]. In addition, two smaller investigations found higher PPARγ expression in malignant versus benign tissues [12,13]. When taken all these studies together, these findings strongly suggested that PPARγ is not a tumour suppressor and that its activation may play a promotive role in the development of PCa.
Figure 1: Structures of PPARγ1 and PPARγ2. At protein level, PPARγ consists of an activation function 1 (AF-1) region, a variable or Hinge (A/B) region, a DNA-binding domain (DBD). a ligand-binding domain (LDB), and an activation function 2 (AF-2) region. PPARγ2 has an extra of 30 Amino acids.
Using a Sleeping Beauty screen in prostate-specific Pten/ mice,
Ahmad et al. identified PPARγ as a new gene that promoted prostate
carcinogenesis [9]. In comparison to littermate controls, mice
having insertions upstream of the PPARγ gene that increased PPAR
protein expression had lower survival rate with increased lung
and lymph node metastases [9]. In these animals, increased PPARγ
expression was correlated with increasing expression of PPARγ
targeting genes for FASN, ATP citrate lyase (ACYL), and acetylCoA
carboxylase (ACC) [9]. Overexpression of PPARγ promoted cell
proliferation and migration in three PCa cell lines, DU145, PC3, and
PC3M, while siRNA knockdown of PPAR had the opposite effect
[9] Ahmad et al. also discovered a significant positive correlation
between PPARγ levels and PCa grades, as well as a link between low
PTEN expression and poor disease-specific survival in patients with
low PTEN expression [9]. Furthermore, Ahmad et al. also analysed
data from the cBioportal (www.cbioportal.org) and discovered that
the PPARγ gene was amplified in 26% of advanced cancers and that
the enzyme 15lipoxygenase 2 (ALOX15B), an endogenous PPARγ
ligand, which reconstructs 15S hydroxyeicosatetraenoic acid, was
upregulated in an extra 17% of cases [9]. Additionally, over half of
all sequenced tumours expressed one or more of the PPAR target
genes for FASN, ACC, or ACLY, strongly suggested a promotive role
for PPARγ activation in the development and progression of PCa [9].
One of the very first studies to examine the involvement of
PPAR in PCa was motivated by the fact that diets high in omega-3
fatty acids appear to be associated with a reduced incidence of
PCa than diets high in omega-6 fatty acids. One of these fatty acid
metabolites, 15-Deoxy-Δ12,14-prostaglandin J2 (15dPGJ2), is a
particular PPAR activator [14] and was found to have anticancer
activity [15], prompting Butler et al. to investigate if the antitumor
qualities were attributable to PPAR activation [16]. They discovered
that while 15dPGJ2 and other PPARγ activators such as ciglitazone
promoted cell death in three PCa cell lines, PPARα and β ligands
did not. This initial discovery sparked more research on the
usefulness of PPARγ activating ligands in PCa, which revealed that
PPARγ agonists reduced androgen receptor (AR) level and activity
while inhibiting PCa cell growth [17-19]. Furthermore, further
mechanistic studies proved unequivocally that these compounds
had an impact independent of the PPARγ (Figure 1.17). According
to one study, PPAR agonists reduced cell proliferation by promoting
the proteasomal degradation of transcription factor specificity
protein 1. (SP1) [20]. Other studies suggested alternative
mechanisms by which PPARγ agonists inhibited PCa cell growth in a
PPARγ-independent manner, which include the inhibition of BclxL/
Bcl2 functions [21], the inhibition of the CXC chemokine receptor
type 4/CXC motif chemokine 12 (CXCR4/CXCL12) axis [22], and
the inhibition of the AKT signalling pathway [23]. A further study
indicated that PPARγ agonists promoted AR signalling in C42 PCa
cells, and that this was PPARγ dependent [24]. As a result, it is
probable that PPARγ agonists stimulate AR signalling, but their
effects on SP1 or other pathways in some cell types result in indirect
AR suppression and lower PCa cell growth. The role of PPARγ and
its ligands in PCa development can be shown in [Figure 2].
Figure 2: The role of PPARγ and its ligands in PCa growth: PPARγ has an oncogenic role in the development and progression of PCa through both AR-dependent and AR-independent mechanisms. PPARγ agonists were found to reduce PCa cell growth through PPARγ-independent pathways. PPARγ antagonists may be useful in treatment of advanced PCa as well as the prevention of PCa. PPARγ: peroxisome proliferator activated receptor gamma, AR: androgen receptor, SP1: specificity protein 1, and PC: prostate cancer.
Previous work demonstrated that elevated FABP5 levels contributed significantly to malignant progression in castrationresistant PC (CRPC) model cell systems by binding and transferring larger amounts of fatty acids, which stimulated the nuclear receptor PPARγ [26]. It is also demonstrated that treatment of PC3-M cells with PPARγ antagonist GW9662 resulted in a similar inhibition of tumour growth to that observed with a FABP5 inhibitor named dmrFABP5. These results suggested that the inhibitory mechanism of dmrFABP5 is connected to the FABP5-PPARγ-signaling pathway [27]. Experiments proved that the cellular uptake of fatty acids was increased as the increasing malignancy of the tested PCa cells, indicating that increased amounts of fatty acids were taken up by the cancer cells and that at least some of excessive amount of fatty acids was used as signalling molecules to stimulate and thus to activate PPARγ [28]. Chemically synthesized FABP5 inhibitor SBFI- 26 was shown to reduce significantly the amount of fatty acids uptake into PC3M cells. So, it was suggested that SB-FI-26 acts as a competitive inhibitor of fatty acids for FABP5 and thus inhibited the transport of intra- and extracellular fatty acids into the cytoplasm [29]. It was suggested that the competitive inhibition of fatty acid uptake by SB-FI-26 may result in a deacress or discontinuation of fatty acid-induced PPARγ activation. Thus, PPARγ may lose its ability to upregulate downstream cancer-promoting genes (such as VEGF), or to downregulate downstream tumour-suppressor genes (such as apoptotic genes) [27, 28]. Previous investigation demonstrated FABP5-PPAR-VEGF signalling axis, rather than the AR-initiated pathways, is the predominant pathway for malignant signal transduction in CRPC cells [27]. Therefore, PPARγ seems to play a critical role in this axis. Interestingly, the bio-inhibitor dmrFABP5 was much stronger than the chemical inhibitor SBFI- 26 in suppressing CRPC developed in nude mice, unlike SBFI- 26, dmrFABP5 did not have an inhibitive effect in cellular fatty acid uptake [28].
Because PPARγ agonists reduce AR activity and PCa cell
proliferation, it was initially assumed to be tumour suppressors in
prostate cells [30-34]. But, PPARγ agonists, on the other hand, were
shown to suppress cell growth and AR activity in an independent
manner of PPAR activity [35-37]. In addition, PPARγ expression also
rises with PC grade/stage [38-40], indicating that the opposite to
the initial assumption is true. In order to study PPARγ as a functional
target in PCa, it is vital to notice the fact that PPARγ has two
isoforms. PPARγ2 which looks exactly like PPARγ1, but is 30 amino
acids longer than PPARγ1 at the amino terminus. PPARγ1 is found
in many organs, whereas PPARγ2 is found primarily in adipocytes and regulates their differentiation [40, 41]. A recent study by Strand
et al [42]. revealed that the two PPARγ isoforms had significant
variations. In this study, the PPAR gene was first knocked out in
mouse prostate epithelial cells, and then the individual PPARγ1 and
PPARγ2 transcripts were subsequently reintroduced. When these
modified cells were used in a prostate reconstitution assay, it was
discovered that restoring PPARγ1 resulted in the development of
adenocarcinoma, whereas restoring PPARγ2 led to the formation
of benign glands. According to a recent study, in many but not all
local and metastatic malignancies, both isoforms of PPARγ are
expressed in human tissue, with PPARγ1 predominating in PCa
cells. Researchers further show that both PPARγ1 and PPARγ2
are expressed in epithelial cells of isolated benign prostate glands
using IHC and RNA in situ hybridization. These results suggested
that PPARγ1 is more important than PPARγ2 in the malignant
progression of PCa. Indeed, such functional characterisation
tests by Strand et al [42]. clearly suggested that PPARγ1 had an
oncogenic capability in prostate cells while PPARγ2 had a tumour
suppressive property. This conclusion was supported by some
recent studies [28]. In a mouse prostate reconstitution test, Strand
et al colleagues clearly proved that expression of PPARγ1 alone in
benign mouse prostate epithelial cells resulted in the formation of
adenocarcinoma-like tissue, whereas expression of PPARγ2 alone
resulted in a highly differentiated phenotype [42]. In the soft agar
colony formation assay, the addition of PPARγ1, but not PPARγ2,
boosted the proliferation of BPH1 cells. It was also discovered that
inhibiting PPARγ1 reduced the proliferation of PCa cell lines with
endogenous or constitutive expression of PPARγ1. This supports the
concept that PPARγ1 is an oncogene. The introduction of PPARγ2
inhibited the proliferation of LNCaP cells, further supporting the
idea of PPARγ2 as a tumour suppressor.
The functional role of PPARү played in malignant progression
of PCa cells has been a controversial issue for quite a long time
[4]. Recent functional characterisation of PPARү isoforms greatly
facilitated the clarification on the functional roles of this gene.
However there still are many issues requiring further study on
PPARү’s functional role in PCa and the underlying molecular
mechanisms. The recent encouraging advances in more reliable and
efficient gene-editing techniques, such as crispr/cas9, provided
better methods to evaluate functional role of PPARү and will help
the research community to understand this gene better and to
develop better strategies for therapeutic interventions.
BTA is supported by a PhD studentship from the Faculty of Medicine, Northern Borders University, Saudi Arabia. GH and YK are supported by a joint International Cooperation Grant from the Science and Technology Department, the Government of Sichuan Province, China (Grant Number: 2019YFH0054).
The authors declare no conflict of interest.
Bio chemistry
University of Texas Medical Branch, USADepartment of Criminal Justice
Liberty University, USADepartment of Psychiatry
University of Kentucky, USADepartment of Medicine
Gally International Biomedical Research & Consulting LLC, USADepartment of Urbanisation and Agricultural
Montreal university, USAOral & Maxillofacial Pathology
New York University, USAGastroenterology and Hepatology
University of Alabama, UKDepartment of Medicine
Universities of Bradford, UKOncology
Circulogene Theranostics, EnglandRadiation Chemistry
National University of Mexico, USAAnalytical Chemistry
Wentworth Institute of Technology, USAMinimally Invasive Surgery
Mercer University school of Medicine, USAPediatric Dentistry
University of Athens , GreeceThe annual scholar awards from Lupine Publishers honor a selected number Read More...