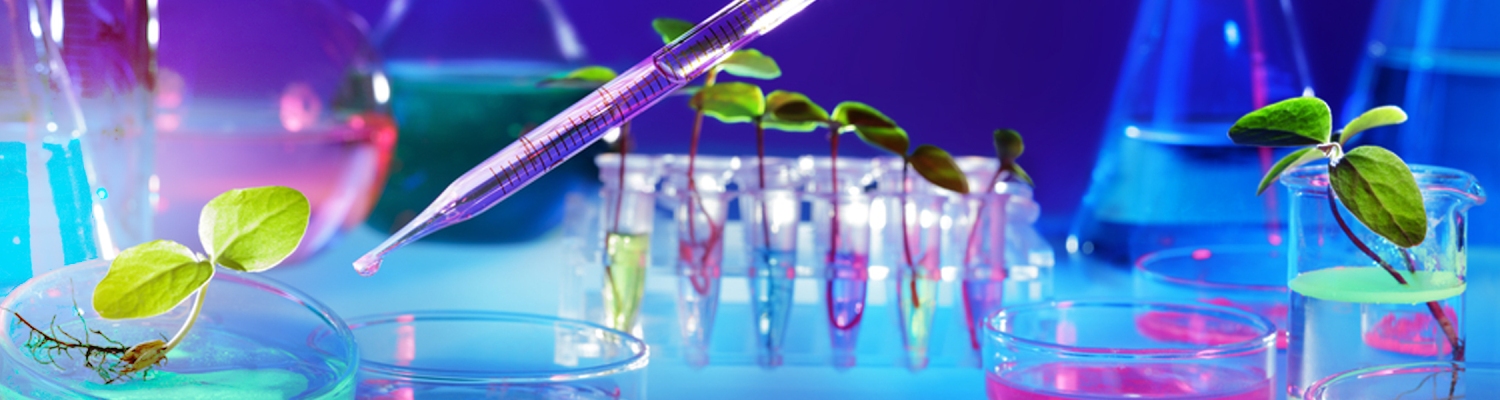
Dirk Roos*
Received:July 29, 2021 Published:August 4, 2021
*Corresponding author:Dirk Roos, Sanquin Blood Supply Organization, and Landsteiner Laboratory, Amsterdam University Medical Center, location AMC, Amsterdam, The Netherlands
DOI: 10.32474/CTBM.2021.02.000135
Chronic granulomatous disease is an immunodeficiency mainly affecting children, usually at an early age, and presents as a susceptibility for severe infections with bacteria, fungi and yeasts. Patients with chronic granulomatous disease have mutations in the genes that encode the components of the leukocyte NADPH oxidase. This system generates reactive oxygen species that are essential for intracellular killing of microorganisms. In a world-wide cooperative action, all published and unpublished mutations in these genes have recently been collected. In addition, known polymorphisms in these genes have also been retrieved, thus aiding in correct interpretation of genetic analysis of the patients.
Keywords: Chronic granulomatous disease (CGD); Mutations; Updates; NADPH oxidase
Chronic granulomatous disease (CGD) is a rare disease, with an estimated incidence of about 1: 250,000 newborns [1]. The patients suffer from severe, recurrent, often life-threatening infections of the lungs, the skin, the gastrointestinal tract or in deep-seated structures like liver, bones, kidneys or brain, as well as in the lymph nodes draining these sites. Infecting organisms include a variety of bacteria and fungi, such as Staphylococcus aureus, Aspergillus species, Klebsiella species, Burkholderia cepacia, Serratia marcescens and Salmonella species [1,2]. In healthy individuals, these microorganisms are killed by phagocytic leukocytes, primarily by neutrophils. These cells contain an enzyme that generates reactive oxygen species (ROS), and together with proteolytic enzymes released from intracellular granules, this creates a very toxic environment for ingested pathogens. CGD patients, however, lack this ROS-generating enzyme, called NADPH oxidase, and are unable therefore, to efficiently kill these pathogens. Treatment of CGD patients consists of aggressive antibiotic and antifungal therapy, often followed by prophylactic treatment. Surgical drainage of infected lymph nodes and abscesses may be necessary. Cure can only be obtained by bone-marrow transplantation. For more details see [1,2].
The leukocyte NADPH oxidase is a complicated enzyme. It consists of five protein components, of which two form a heterodimer embedded in the plasma membrane of the phagocytic leukocyte and the other three form a heterotrimer in the cytoplasm [3,4]. In this situation, in resting cells, the enzyme is not active. However, upon phagocytosis of microorganisms, the cytosolic trimer attaches to the membrane dimer, thus forming a complex of five proteins. This complex formation is accompanied by structural changes that allow NADPH in the cytosol to donate electrons to the system. These electrons are then transported within the enzyme to the apical side of the plasma membrane, i.e. to the inside of the phagocytic vacuole (phagosome) that contains the ingested microorganism (Figure 1). Here, the electrons combine with molecular oxygen, thus generating superoxide (O2‒), which subsequently reacts with protons to hydrogen peroxide (H2O2). These reactive oxygen species are thus formed in close contact with the ingested microorganisms, and – together with proteolytic enzymes released from azurophilic granules – kill the ingested microbes.
Anywhere in this whole process things can go wrong. The plasma-membrane dimer consists of gp91phox (glycoprotein of 91kDa; phox for phagocyte oxidase) and p22phox. Gp91phox (also called Nox2) is the enzymatic subunit, p22phox is needed for stable expression of gp91phox. Mutations in the CYBB gene encoding gp91phox is the most common cause of CGD (about two-thirds of all cases). Mutations in CYBB may result in absence of gp91phox (X910), diminished presence of gp91phox (X91‒) or normal expression of an inactive form of gp91phox (X91+). Each of these phenotypes will cause CGD, although the X91‒ form (with some enzymatic activity preserved) may present with milder clinical symptoms than the other two forms. Since CYBB is located on the X chromosome, this form of CGD is X-linked, and the vast majority of the patients are therefore boys. However, girls with a CYBB mutation on one of their X chromosomes can have a skewed distribution of cells with the mutated X chromosome inactivated and cells with the wild-type X chromosome inactivated. In case very few cells have an active wildtype X chromosome, such girls may present as CGD patients.
All other NADPH oxidase components are encoded by autosomal genes. Therefore, CGD caused by mutations in these genes affect boys and girls in equal numbers. Often, such patients have the same mutation on both autosomes, especially in countries with a high ratio of parental consanguinity. In such countries the number of patients with autosomal CGD may even exceed that of X-CGD patients.
Diagnosis of CGD is based on clinical presentation, reaction to treatment and family history, but for certainty must be followed by laboratory investigations showing the lack of ROS formation by the patient’s neutrophils, as described in [3,4]. To be able to offer genetic advise to the family, to perform prenatal diagnosis and eventually to choose a suitable bone marrow donor, genetic analysis is absolutely necessary. However, it is sometimes difficult to conclude that a certain genetic variation found in a patient is the cause of his or her disease. Therefore, a list with all mutations found in proven CGD patients is helpful in making this choice. Also a list with proven polymorphisms in the CGD genes aids in correct interpretation of the results. Both actions have recently been updated in a world-wide effort involving more than twenty medical centers [5,6]. For this purpose, the literature was screened and the authors of the new updates have contributed their unpublished observations. For X-CGD this was the fourth update, the previous one dating from 2010. For autosomal CGD this was the third update, also since the second in 2010.
The list of X-CGD mutations contains more than 1000 different mutations observed in almost 3000 patients [5]. Many new patients from China and India were added. If known, the list also indicates the effect of the mutations on the expression of gp91phox, and for each mutation the presence of female heterozygous patients. In addition, for each mutation the number of CGD families and patients is given. This last item enables analysis of the frequency of each mutation, and therefore identification of certain hotspots in the mutational landscape. Also indicated are the type of mutation per patient, i.e. deletion, insertion, insertion-deletion (indel), missense, nonsense, or splice site mutation. In X-CGD, the CYBB deletions are the most common variation, followed by splice site and missense mutations.
The list of autosomal CGD mutations includes mutations in CYBA (encoding p22phox), in NCF1 (encoding p47phox), in NCF2 (encoding p67phox) and in NCF4 (encoding p40phox); these last three genes encode the three cytosolic components of the NADPH oxidase [6]. All together this includes another 240 different mutations in about 1800 CGD patients. The p47phox component deserves special attention, because the NCF1 gene is accompanied on each side by a pseudogene with which it easily exchanges information. This results in the most frequently encountered CGD mutation, viz. a GT deletion in NCF1 causing the absence of the p47phox protein. Although this results in a rather mild form of CGD (because some NADPH oxidase activity is still present), genetic detection is difficult owing to the presence of the pseudogenes.
In addition, a few more genetic mutations have been added to the new updates, because recent information has resulted in the recognition of some genes which, when mutated, give rise to CGD-like symptoms. First, for NADPH oxidase activity, sufficient substrate (NADPH) must be present. In neutrophils, NADPH is largely generated in the glycolytic pathway, with glucose-6- phosphate dehydrogenase as a key enzyme. Thus, some G6PD mutations, resulting in very low NADPH levels in neutrophils, lead to low NADPH oxidase activity and as a consequence to CGD-like symptoms. These mutations are included in the X-CGD mutation update [5]. We have to realize, however, that not all variations in G6PD will lead to problems in the defense against pathogens: only those variations that cause a strong decrease in G6PD activity in neutrophils will have that effect, and possibly variations in other genes may also be required for that effect.
Further, CYBC1 is a gene that encodes cytochrome b558 chaperone-1, a protein that is needed for maturation and full expression of gp91phox [7]. Two mutations in this gene have been discovered that cause a low expression of gp91phox and CGD as a result. Finally, the process of NADPH activation during phagocytosis of microorganisms by neutrophils is under control of the small GTPase protein RAC (RAC2 in neutrophils, RAC1 in macrophages) (Figure 1). One mutation in the RAC2 gene is known to disturb this activation process and thus to cause CGD-like symptoms [8]. Since RAC2 is involved in a number of different processes in leukocytes, other variations in the RAC2 gene cause other problems, such as common variable immunodeficiency (CVID) or severe combined immunodeficiency (SCID). The abnormalities in CYBC1 and RAC2 that do lead to CGD-like symptoms have been added to the new autosomal CGD mutation update [6].
In summary, these two publications [5,6] contain valuable information for clinicians taking care of CGD patients and for investigators studying the properties of the leukocyte NADPH oxidase in health and disease. With these two updates, CGD may well be the best characterized immunodeficiency.
CGD- Chronic Granulomatous Disease; CVID- Common Variable Immunodeficiency; CYBA- Cytochrome-B Alpha Subunit; CYBBCytochrome- B Beta Subunit; CYBC1- Cytochrome b558 Chaperone-1; G6PD- Glucose-6-Phosphate Dehydrogenase; gp- glycoprotein; H2O2- Hydrogen Peroxide; indel- insertion-deletion; kDa- kilodalton; NADPH- Nicotinamide-Adenine-Dinucleotide Phosphate, Reduced, NCF- Neutrophil Cytosolic Factor; Nox- NADPH oxidase; O2‒ Superoxide; p-protein; phox- phagocyte oxidase; RAC- Ras-related C3 Botulinum Toxin Substrate; ROS- Reactive Oxygen Species; SCIDSevere Combined Immunodeficiency; X-CGD, X-linked CGD.
Bio chemistry
University of Texas Medical Branch, USADepartment of Criminal Justice
Liberty University, USADepartment of Psychiatry
University of Kentucky, USADepartment of Medicine
Gally International Biomedical Research & Consulting LLC, USADepartment of Urbanisation and Agricultural
Montreal university, USAOral & Maxillofacial Pathology
New York University, USAGastroenterology and Hepatology
University of Alabama, UKDepartment of Medicine
Universities of Bradford, UKOncology
Circulogene Theranostics, EnglandRadiation Chemistry
National University of Mexico, USAAnalytical Chemistry
Wentworth Institute of Technology, USAMinimally Invasive Surgery
Mercer University school of Medicine, USAPediatric Dentistry
University of Athens , GreeceThe annual scholar awards from Lupine Publishers honor a selected number Read More...