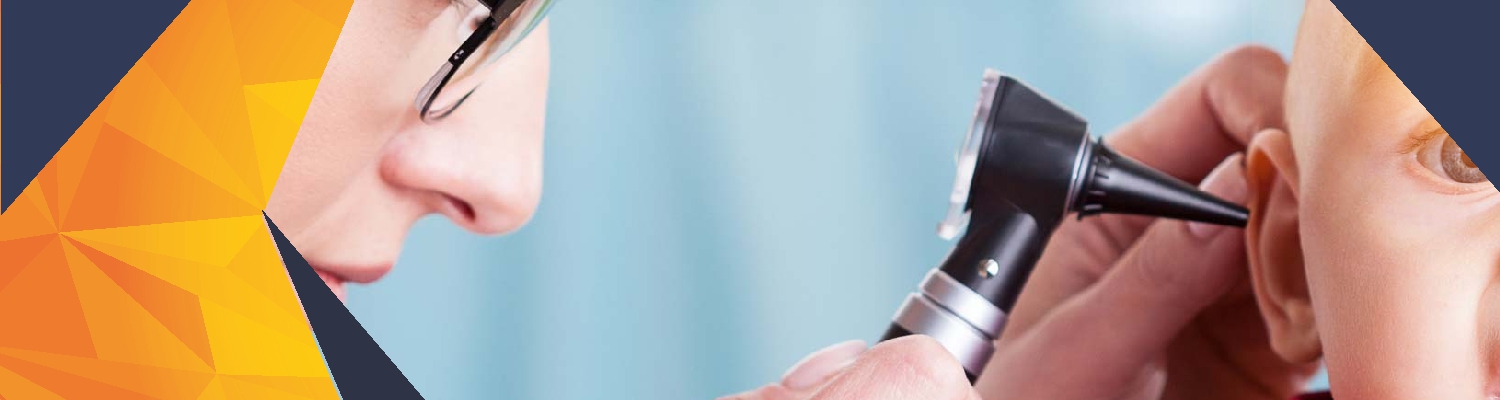
ISSN: 2641-1709
Jan Myjkowski*
Received: October 18, 2022; Published: November 02, 2022
Corresponding author: Jan Myjkowski, Otolaryngology Outpatient Clinic, Mielec, Poland
DOI: 10.32474/SJO.2022.09.000308
According to the Georg von Bekesy’s theory of traveling wave, announced in 1928, a sound wave from the eardrum travels to the receptor through the middle ear, cochlear fluids, basilemma and bending of the acoustic cell hairs. An analysis of the mechanisms of transmission of information indicates a large number of problems related to the conveyance of a sound signal traveling this way. Experimental studies corroborate some objections which regard as follows:
a. Threshold hearing according to this theory, which is impossible due to wave energy disappearance.
b. Impossible is a resonance of very short audible sounds.
c. Inertia in the ear, which creates problems in the case of high frequencies.
d. Resolution of the lowest frequencies according to the wavelength.
e. Calculation of the basilemma’s proper vibrations in line with Bekesy’s theory.
f. Difference in the wave speed in cochlear fluids and the basilemma.
g. Amplification of quiet tones, especially multitones.
h. Time for generating a receptor potential
i. Lack of transmission of high frequencies after stapedotomy.
j. Immobilization of the basilemma in a cochlear implant, which does not affect the hearing.
k. Mechanism of converting the sound wave energy into the receptor potential.
l. In total, those ambiguities related to the traveling wave theory have led to a new theory of hearing under the name ‘Submolecular Theory of Hearing’. The difference consists in acknowledging another signal path to the receptor, accepting the molecular mechanisms of generating receptor potential without tip-links and amplification of quiet sounds through intercellular transformations.
Keywords: Inertia; resonance; receptor potential; basal membrane
The loudest signal ever emitted on the Earth is the explosion of Krakatoa volcano in 1883.The explosion rang within a 4,300 km radius. At a distance of 64 km, the sound wave energy caused tympanic membrane ruptures in seamen. The receptor’s reaction to a sound depends upon the sound intensity, viz. the sound wave amplitude, signal frequency and its duration time and the receptor’s sensitivity itself. A minimum hearing level for a 1,000 Hz tone, its pressure being 2 x 10 -5 Pascal, in a young person corresponds to a wave whose amplitude amounts to 8 picometres (pm) in the external auditory meatus (‘eam’) =0 dB. There are cases of hyperacusis, where a receptor can receive sounds under 0 dB. Then, there are also creatures receiving considerably quieter sounds with various frequencies. A barn owl has a hearing threshold on a level of 1 pm [1]. It is not possible to generate a traveling wave on the basilemma with an amplitude 130 times smaller than the oxygen atom diameter. The amplitude of a sound wave in the middle ear diminishes, whereas in cochlear fluids its reduction is considerably higher. If a sound of such an amplitude is audible, this means that the sound reaches the receptor on another path, not through cochlear fluids nor the wave traveling on the basilemma. So, the only possible path for the signal to the receptor is the osseous cochlear housing.
Sound wave energy from the space is received through the basilemma. Its purpose is the absorption of sound wave energy and its conveyance to the middle ear ossicles. The basilemma’s structure in various quadrants is diversified, which influences the reception and transmission of energy. Diversified is also the tympanic membrane’s position towards the wave incidence. Examined was the speed and translocation of the tympanic membrane in various places. It was noted that for the same wave, the amplitude of vibrations of the posterior quadrants was higher than in the case of the anterior ones. The difference in the displacement on both the side of the malleus causes its swinging (rocking) rotary motion which is conveyed to the incus and a spherical incudostapedial joint. The rotary motion of the malleus depends on the wave energy and sound wave frequency is converted into the rocking motion of the stapes [2]. As the sound intensity and frequency increase, so reduced is the piston-like motion; instead, increased is the movement of the stapes lamella, respectively, in the horizontal axis, and in the longitudinal axis in the case of higher frequencies. In the case of rocking movements of the stapes, the full range of frequencies will reach the receptor. It is proved by our hearing up to 20 kHz, whereas other mammals can hear up to 100 or even 200 kHz. Stapedotomy does not ensure rocking actions of the stapes; only the transmission of low frequencies of the mechanism of the stapes piston. High frequency signals will not reach the receptor on a path where vibrating elements on this path are subject to the inertia law.
The sound wave energy from the basilemma is conveyed to cochlear fluids through the middle ear ossicles. In amphibians, reptiles and birds there is one middle ear ossicle formed into a small column which connects the basilemma with the oval window. Instead, in mammals there are three ossicles, which has double importance. Firstly, the weight of the ossicles is important in the inertia of the vibrating parts in the middle ear. Inertia in the wave motion is calculated from the formula:
(2 π x frequency)2 x amplitude x mass g x mm/s2
Secondly, the length difference between the malleus and the long limb of the incus constitutes the lever mechanism [leverage], crucial mainly for low frequencies. The ratio of the arms of the malleus: incus is 1.3 :1. That is why the wave amplitude conducted through the ossicles is reduced at a ratio 1.3 : 1. If a sound wave under testing in the auditory meatus amounts to 90 dB, so after conversion into the wave amplitude the wave will be 500 nm. The 0 dB threshold wave in the auditory meatus has an amplitude of 8 picometres (Resnick, Halliday). In the first case the stapes is subject to a wave with an amplitude of 384.6 nm. Instead, in the vase of the threshold tone the value is 6.15 picometres (pm).
Observations of vibrations of the stapes with a doppler laser vibrometer have proved that (Asai 1999, Huber 2003, Kwacz 2013) the values of vibrations of the stapes depends upon the intensity and frequency, too. It diminishes pro rata to the increase in the sound frequency [3]. For low frequencies - under 1,25 kHz (90 dB), the amplitude of stapes’ vibrations amounts to 8.53 x 10-9 m. For a frequency of 4 kHz ,the amplitude is 7.53 x 10-10 m. Eventually, for 10 kHz, the amplitude is 1.17x10-10 m (Kwacz, Gambin). According to the results available from several research establishments, for a sound wave amplitude in the external auditory meatus - 90 dB = 500 nm, examined upon the stapes the value is [4].
a) For a frequency of 1250 Hz 8.53 nm and will diminish 58.5 times from the external auditory meatus.
b) For a frequency of 4,000 Hz , it amounts to 0,75 nm and is reduced 666 times. For a frequency of 10 kHz, it amounts to 0,11 nm and is reduced 4,300 times.
c) It is hardly believed that an acoustic signal might be transmitted to the receptor this way.
In line with Bekesy’s traveling wave theory, the sound wave energy is transmitted to cochlear fluids. According to the methodology given by the author of the traveling wave theory calculated was the wave amplitude at the beginning of the vestibular meatus and nearby the cupula (Gambin).
a. For a wave of 1,250 Hz, the 90 dB wave amplitude is 0.179 nm. For the 90 dB wave amplitude, the value nearby the cupula is 0.372 nm.
b. For a 4,000 Hz wave, the 90 dB wave amplitude at the beginning of the meatus is 0.088 nm. For a 4,000 Hz wave, the 90 dB wave amplitude nearby the cupula is 0.018 nm.
c. For a 10 kHz wave, the 90 dB wave amplitude at the beginning of the meatus is 0.000874 nm. For the 90 a 10 kHz wave, the 90 dB wave amplitude nearby the cupula is 0.00181 nm.
It is puzzling that according to those investigations, the sound wave amplitude, irrespective of the frequency, will increase by around 100% on the way from the beginning of the vestibule towards the cupula. As perplexing is a fall in the amplitude of a 500 nm wave in the external auditory meatus (1000 Hz) - down to 11.7 nm - vibrations of the stapes’ and 0.275 nm at the beginning of the vestibular duct. This is yet more conspicuous for 10 kHz: external auditory meatus - 500 nm, stapes lamella: 0.117 nm and initial wave in cochlear fluids: 0.000874 nm. For 10 dB and 10 kHz, the wave amplitude in the vestibular duct at the base is 0.0000000874 nm (Gambin). This implies an error in the assumptions of the traveling wave theory. Sounds with intensities of 10 dB and frequencies of 10 kHz are audible to us although the amplitude of this wave on the way through cochlear fluids disappears 230 million times even before reaching the receptor. This is physically impossible, so there must be another signal path without such losses of energy and time as well. Susceptibility to displacements of the oval window, which is twenty times smaller than that of the round window, in conjunction with the resonance of the traveling wave in cochlear fluids and proper vibrations of the basilemma will generate a traveling wave on the basilemma. There are some objections to the feasibility of the basilemma’s spontaneous vibrations and the calculated proper vibrations since the basilemma is loaded from the top by the organ of Corti and a band of connective tissue on the inferior membrane surface. In addition, the whole is immersed in cochlear fluids. Resonance of the longitudinal sound wave in cochlear fluids with the transverse wave traveling on the basilemma is difficult to occur due to a very big difference in the speed of both the waves [5].
A sound wave can travel in a fluid at a speed of 1,450 m/s, whereas the speed of a traveling wave depends upon the wave frequency, and for 2-3 kHz this value is 1.9-2.4 m/s, while for frequencies 29-40 kHz - the speed of the wave traveling forwards is 9-8 m/s [6]. Therefore, the average speed of a traveling wave is 290 times smaller than the speed of a sound wave traveling in cochlear fluids. Moreover, resonance is actually unlike to occur at low frequencies when the wavelength is many times longer than the basilemma’s length. Resonance is unlikely to occur, too, in the case of tones with duration times of tenth parts of ms which are physiologically audible [7]. A signal will reach the receptor, but not through cochlear fluids and resonance [8]. As Bekesy has it, a wave traveling on the basilemma will lead to a reduction and increase of the distance between the basilemma and the covering membrane, whose consequence is a flow of cochlear fluids and bending of acoustic cell hairs [9]. It is not a stationary, laminar flow. If encoded information should be conveyed this way, therefore the motion of fluids must comply with the sound amplitude and frequency, too. Since the organ of Corti vibrates as the basilemma does, it is provided with mass, speed and acceleration, and thus will be subject to the inertia law which limits the conduction of high frequencies. On the inferior pole of OHCs arranged are delicate efferent and afferent synapses, susceptible to continuous vibrations of OHCs with the organ of Corti and the basilemma. In a wave motion, the fluid in the middle ear, set agoing by a traveling wave has a speed, acceleration and mass – so it is subject to inertia. If the amplitude od’s a 90 dB and 10 kHz wave in the auditory meatus, of an amplitude of 500 nm, diminishes in the initial section of the vestibular duct down to 0.8 pm, a traveling wave and ‘flows of fluids’ bending the hairs of thicker acoustic cells 125,000 times are unlikely to be generated.
It should be also noted that the receptor is reached as well by a 0 dB signal whose amplitude is 8 pm in the external auditory meatus which reduces the amplitude on the way through fluids and the basilemma more than 6 million times – in line with the calculations made according to the traveling wave theory. The cause of such calculations may be the straightening of the cochlea up to a straight tube and a connection of the vestibular duct with the cochlear duct so that the wave may travel on both the cochlea’s sides and an incorrect calculation of the cochlea’s proper vibrations by assuming that the basilemma is an independent element vibrating in the air. The time for generating receptor potential is almost indeterminable; nor any latency period is observed (Pruszewicz). This results from the speed of sound transmission through a bone – approx. 4,000 m/s as well as from a short signal path to the receptor. A cochlear implant, in the case of partial deafness, corroborates the thesis that the basilemma does not play the role attributed thereto by the theory of traveling wave. Stapedotomy procedures indicate there is neither any transmission nor amplification of high frequencies when operates only the piston conveying wavs to cochlear fluids [10,11]. Any and all problems related to the path on which information is transmitted to a receptor through cochlear fluids and the basilemma will give a strong foundation to set forth a thesis there is another path conveying a signal to the receptor. This is the signal path from the middle ear through the cochlear osseous housing directly to the receptor. The energy of sound waves arrives at the ear incessantly event at night. It can be either stored or destroyed. In line with the 1st principle of thermodynamics it can be turned into another form of energy. That is why the information in the form of energy encoded in a sound wave reaches the receptor unchanged; instead, the other part of energy will be subject to degradation while traveling through cochlear fluids when the wave amplitude diminishes millions of times.
Molecular mechanisms are responsible for the reception and processing information in an acoustic cell [12]. When the signal is not received by the receptor, mechanical amplification through pulling at the basilemma by contracting OHCs is not possible. There is a perfect intracellular amplification of the received signal, too weak to reach the center. Such an amplification is present in other sense organs [12]. Mechanical amplification causes problems to multitone with both loud and quiet tones and numerous harmonics. A mechanical amplification of quiet tones is time consuming and brings about a separation of information for the center. Unamplified information is sent directly. After amplification, amplified information is sent to the center in a considerable delay with other information pertinent to other waves. Bearing in mind all problems related to the transmission of auditory information to the receptor through cochlear fluids and the basilemma it is certain there is another signal path to the receptor. In this process involved is the conduction of the cochlear housing bones and the ‘osseous boneless conduction, viz. conduction of sound waves through soft tissues, which, apart from rocking movements of the stapes, ensures direct transmission of sound waves from the middle ear to the housing bone of the labyrinth. A proof for the conduction of sound waves through soft its issues is the hearing of a baby in the mother’s womb from the second half of the gestation. The baby can hear its mother’s voce, remembers the voice after birth, which implies the recognition and remembering the voice timbre. A sound wave does not have any mass, and thus it is not subject to the inertia law. High frequencies may be freely transmitted in the man directly to the receptor in the range of up to 20 kHz. In other creatures’ high frequencies arrive directly the receptor and are received in a range of up to 200 kHz [in a bat] and as a record - of up to 300 kHz in a greater wax moth Galleria mellonella).
Bio chemistry
University of Texas Medical Branch, USADepartment of Criminal Justice
Liberty University, USADepartment of Psychiatry
University of Kentucky, USADepartment of Medicine
Gally International Biomedical Research & Consulting LLC, USADepartment of Urbanisation and Agricultural
Montreal university, USAOral & Maxillofacial Pathology
New York University, USAGastroenterology and Hepatology
University of Alabama, UKDepartment of Medicine
Universities of Bradford, UKOncology
Circulogene Theranostics, EnglandRadiation Chemistry
National University of Mexico, USAAnalytical Chemistry
Wentworth Institute of Technology, USAMinimally Invasive Surgery
Mercer University school of Medicine, USAPediatric Dentistry
University of Athens , GreeceThe annual scholar awards from Lupine Publishers honor a selected number Read More...