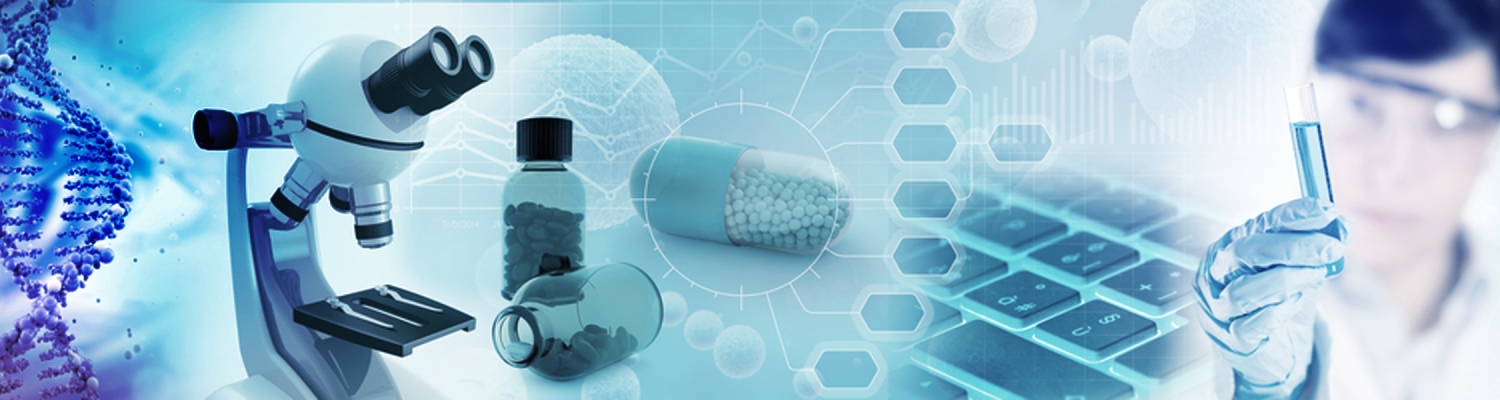
ISSN: 2641-1725
Peng Cheng1,2,3, Hai-Yang Liao1,2,3 and Hai-hong Zhang1*
Received: December 21, 2021 Published: January 24, 2022
*Corresponding author: Hai-hong Zhang, The Second Clinical Medical College, Lanzhou University, China
DOI: 10.32474/LOJMS.2022.06.000230
Osteosarcoma (OS) is the most common bone malignancy in children and adolescents. Despite the efficacy of multiscientific and multimodal therapies for OS, the prognosis of OS is not satisfactory, which highlights the need to find more effective therapies. The NF-κB pathway is a highly complex and unique intracellular signaling pathway, whose abnormal expression has been implicated in a variety of human diseases, including cancer, spinal cord injury, degenerative disc changes, traumatic brain injury, and tuberculosis. NF-κB pathway is considered to be a common signaling pathway in human cancer. Multiple studies have shown that this pathway is often abnormally expressed in OS and regulates the pathophysiological processes of osteosarcoma, such as gene expression, cell proliferation and apoptosis, invasion, migration and tumor angiogenesis. Therefore, manipulation of the expression or function of the associated NF-κB pathway may be a potential clinical target for treating OS. In this review, we summarize the characteristics of the NF-κB signaling pathway and its role in the pathogenesis and development of OS, as well as the current research mechanisms of compounds that inhibit or target the NF-κB pathway in OS
Keywords: NF-κB Pathway; Osteosarcoma; Tumogenesis; Therapeutic Target; Apoptosis
Osteosarcoma is the most common malignant bone tumor
affecting children and adolescents worldwide [1], Osteosarcoma
is a very aggressive malignant tumor, and it has features of
local aggressive growth and a high metastatic potential [2,3].
At present, the treatment of OS mainly includes surgery,
radiotherapy, neoadjuvant chemotherapy and postoperative
adjuvant chemotherapy. Although there are still risks of metastasis,
recurrence and chemotherapy resistance under the current
multi-science and multi-mode treatment, their outcomes are not
satisfactory and the 5-year overall survival rate of affecting patients
is estimated at approximately 25% [4]. Patients with distant
metastases have a poorer prognosis, with a 5-year survival rate of
only 20% [5]. More importantly, the mechanisms of osteosarcoma
progression are complex, and the molecular mechanisms of
etiology and pathogenesis remain almost unknown. Therefore,
novel treatment strategies are urgently required to improve the
outcome and quality of life of patients with osteosarcoma.
Despite the fact that the etiology of OS is not fully elucidated,
there has been a large body of evidence that the disease is involved
in dysregulation of multiple intracellular signaling pathways,
especially the NF-κB pathway. NF-κB pathway is a series of events
that play a key role in a variety of physiological and pathological
processes. Such as inducing immune and inflammatory responses
and regulating apoptosis [3,6-10]. This pathway is also related
to the occurrence and development of various diseases and
pathological mechanisms, such as myocarditis, spinal cord injury,
degenerative disc changes, traumatic brain injury and tuberculosis
[11-15]. In addition, aberrant NF-κB signalling is observed in, and
often a contributing factor to, many human diseases, including
autoimmune disorders, chronic inflammatory diseases, and cancer
[16-18]. It has been reported that the NF-κB signaling pathway is
an important oncogenic pathway in human cancers. Dysregulation
of this pathway is associated with many types of malignancies, such
as prostate cancer, colorectal cancer, bladder cancer, breast cancer
and osteosarcoma [19-23]. Emerging evidence has indicated that
the NF-κB signaling pathway plays central roles in the control
of cell growth, apoptosis, stress response, and several other
physiological processes [8,24,25]. It protects tumour cells from
apoptosis, while also supporting cell invasion, metastasis, and
angiogenesis of tumour cells [26,27]. In addition, the activation
of the NF-κB pathway has been confirmed as an essential step towards EMT in tumor cells. It has been reported that the NF-κB
pathway is overactivated in osteosarcoma, resulting in excessive
proliferation of tumor cells and accelerated development of OS [28].
In addition, abnormal expression of this pathway is widely involved
in cell processes such as proliferation, apoptosis, apoptosis, cycle,
chemotherapy resistance, and metastasis of tumors, including
osteosarcoma [29,30]. At present, a large number of studies
have confirmed that NF-κB pathway is an important molecular
mechanism in the pathogenesis and development of OS, and several
compounds targeting NF-κB pathway have been synthesized
to block the pathogenesis of OS at the molecular level, which is
expected to improve the survival rate of OS patients. In this review,
we briefly summarize the NF-κB signaling pathway, and then focus
on the pathogenesis significance of this complex pathway in OS, the
effect of crosstalk between NF-κB and other signaling pathways on
OS, and the potential significance of NF-κB signaling pathway in OS.
The NF-κB protein forms a protein family homologous to the chicken oncogene REL and was originally described as a B cellspecific factor that binds to a specific DNA site (10-base pair motif) in the intron enhancer of the immunoglobulin κ light chain gene [31]. There are five known NF-κB subunits in mammals, each characterized by anchor protein repeat elements: P65 (RelA), RelB, REL (C-REL), P50/ P105, and P52/P100. NF-κB is the prototypical dimer transcription factor of the REL protein family. NF-κB protein has an n-terminal domain of about 300 amino acids, called the Rel Homology (RH) domain, which is required for dimer, specific DNA sequence binding, and interaction with IκB inhibitors [32,33]. The Rel protein family is divided into two groups based on differences in structure, function and synthesis. The Rel protein family is divided into two groups according to their structure, function and synthesis. The first group consists of P50 (NF-κB1) and P52 (NF-κB2), which are synthesized as 105 and 100 KDa precursors respectively, while the second group consists of P65 (REA), Rel (C-REL), RelB and Drosophila Rel proteins with Transcriptional Activation Domains (TAD). They are not synthesized as precursors [34,35] (Figure 1). They function as dimers in two separate but interconnected branches of the NF-κB pathway: canonical and atypical) (Figure 2). NF-κB dimers are present in the cytoplasm of most mammalian cells in inactive form as a result of binding to specific members of the NF-κB inhibitory protein IκB family. There are several known members of the IκB family, including IκBα, IκBβ, IκB MIDI, Bcl-3, P100, and P105, all of which contain anchor protein-like repeats that mediate binding and inhibition of NF-κB [36]. Activation of NF- κB occurs in most cells in response to a variety of stimuli including cytokines, immunomodulators, and other stresses. Activation of NF-κB requires the release of molecules from its inhibitory IκB, which is initiated by a variety of stimuli and conditions involved in immune function and development. The main activators of NF- κB include RECEPTOR Activator of NF-κB ligand (RANKL), TNFα, lymphotoxin, bacterial endotoxin, Toll-like receptor, TLR ligand, CD40L, Interleukin-1 (IL-1) and oxygen free radical [37]. The normal pathway of NF-κB activation is initiated by inflammatory cytokines, including IL-1β, and usually leads to the activation of complexes containing RelA or cRel [38]. However, NF-κB activation involves multiple transduction pathways, most of which depend on the phosphorylation and degradation of IκB [35,39]. NF-κB is responsible for transcription and expression of a series of genes encoding cytokines, enzymes, apoptosis, proliferation and adhesion molecules that are involved in inflammation and tumorigenesis [40]. In vitro studies have shown that NF-κB binding level in human osteosarcoma cell culture is significantly increased compared with normal bone cells [41]. Other studies have found that at least 75% of patients showed positive staining for the activated NF-κB pathway, and the median overall survival time of patients with NF-κB active osteosarcoma was shorter than that of patients with NF-κB active osteosarcoma [42]. Therefore, we determined that NF-κB plays an important role in many aspects of osteosarcoma development.
Figure 2: NF-κB signaling pathways. (A) Canonical NF-κB receptors TNFR, IL-1R and TLR are activated by their respective ligands, resulting in IKKβ activation. CRC biomarkers such as IL-6, IL-1 and TNFα can enhance this activity. Activated IKKβ phosphorylates IκBα, which causes its subsequent ubiquitination, separation from the p65/p50 complex and proteasomal degradation. p65/p50 heterodimers then translocate to the nucleus and can bind to their respective DNA elements and promote gene transcription. (B) In the noncanonical NF-κB signaling pathway, activation of noncanonical NF-κB receptors, including RANK and CD40, activates NIK, resulting in IKKα phosphorylation. Activated IKKα then phosphorylates p100, causing its polyubiquitination and processing to p52 via proteasomal degradation. The p52/RelB heterodimer then translocates to the nucleus to bind to its respective DNA elements.
As an intracellular signaling pathway, NF-κB signaling pathway plays an important role in the development and progression of various tumors. Nf-κb transcription factors have been reported to be involved in cell proliferation and cell death, and in the context of cell proliferation, they can regulate the expression of A variety of cell cycle modulators, such as Cyclin A, cyclin D1 or cyclin-dependent kinase 6 (CDK6) [43]. In addition, activation of NF-κB is an important step to protect cells from TNF-α-induced apoptosis. In most cases, cancer cells express combined activation of NF-κB and their proliferation is not regulated or insensitive to cell death [44,45]. Besides, the activation of NF-κB pathway is also closely related to tumor angiogenesis, epithelial-mesenchymal transformation, chemical resistance and other pathological processes [25]. Given that osteosarcoma is a disease that exhibits a variety of clinical manifestations and aggressions, NF-κB is an ideal target because its constitutive activation may contribute to multiple steps of transformation, initiation, promotion, angiogenesis, invasion, and metastasis (Figure 3).
Promote the growth, proliferation and growth of tumor cells
The spontaneous growth of cancer cells is usually controlled by changes in the expression of growth factors or growth factor receptors, leading to cell proliferation. Dysregulation of nuclear factor-κB (NF-κB) signaling is associated with excessive cell proliferation and development signaling during tumgenesis. In fact, this pathway has been reported to be involved in inflammatory proliferation and differentiation of osteosarcoma cells [46]. Activation of NF-κB pathway is closely related to the proliferation of osteosarcoma MG63 cells. It was found that the proliferation rate of NF-κB high expression group was significantly higher than that of low expression group. Inhibition of NF-κB activity significantly reduced the proliferation capacity of MG63 [28]. In addition, Huang et al. found that the NF-κB pathway is overactivated in osteosarcoma cells, and its overactivation is beneficial to the proliferation and growth of tumor cells. Inhibition of NF-κB activity in tumor cells by angarin, an antioxidant ellagic tannin found in pomegranate juice, with known anti-proliferation and anti-angiogenic properties, significantly inhibited the proliferation and growth of cancer cells [47]. Other studies found that the expression of NF-κB was up-regulated in OS tissues and cell lines. The content of NF-κB in supernatant of OS cell lines was higher than that of normal cells, and the proliferation of OS with high NF-κB expression was significantly stronger than that of normal cells. Inhibition of NF-κB significantly reduced the proliferation of OS cancer cells [48]. Besides, a large number of studies have reported the role of NF-κB in regulating cancer cell proliferation, including a key role in OS cell proliferation [49-51]. Based on the above evidence, it is not difficult to see that NF-κB signaling pathway plays an important role in the regulation of OS cancer cell proliferation and growth, which provides a new direction for targeting NF-κB pathway in the future treatment of OS.
Apoptosis
Figure 4: NF-κB negatively regulates the JNK/AP-1 axis and participates in TGF-β 1-induced apoptosis. JNK/AP-1 promotes cell death. IKK/NF-κB promotes cell survival.
Apoptosis is a kind of programmed cell death, which is an important process of normal development and tissue homeostasis. Apoptosis is the main mechanism of cell death induced by chemotherapy and radiotherapy in many cancers, including esophageal cancer. NF-κB has also been shown to play a role in regulating apoptotic processes and is a key gene mediator involved in apoptosis. Anti-apoptosis is a common feature of cancer cells and is associated with increased expression of antiapoptotic factors such as Bcl-2 or Bcl-XL or decreased expression, inactivation or mutation of pro-apoptotic factors such as Foxo3a or p53. Interestingly, NF-κB directly regulates an effective antiapoptotic pathway and also activates the expression of several anti-apoptotic genes, including cIAP, TRAF, bcl-2 homolog A1/BF1- 1, 1EX-IL, and Bcl-XL [52] (Figure 4). Recently, Jiang, et al. found that ubiquitination peroxisome proliferator activated receptor α (PPARα) activates NF-κB pathway in OS cells, thereby promoting osteosarcoma cell viability and inhibiting apoptosis [53]. Sorafenib (an oral multikinase inhibitor) reduces the invasion and migration of OS cells, induces NF-κB activation and the expression of an anti-apoptotic protein (Bcl-2) encoded by NF-κB target gene, and reduces the apoptosis of OS cells. Inhibition of NF-κB pathway resulted in the opposite result [54]. These findings suggest that abnormal expression of NF-κB pathway plays an important role in regulating OS, and activation of NF-κB pathway inhibits apoptosis of cancer cells. This allows the targeted NF-κB pathway to eliminate the inhibition of OS apoptosis, so targeting NF-κB may be a potential treatment for OS.
Epithelial mesenchymal transformation and invasion and metastasis
Epithelial Mesenchymal Transformation (EMT) refers to the transformation process that adhesive epithelial cells migrate into mesenchymal cells under certain circumstances. It enables epithelial cells to possess the characteristics of mesenchymal cells. In malignant tumors, EMT is closely related to the invasion and metastasis of tumor cells [55]. On the other hand, osteosarcoma is an interstitial tumor, and patients with a high degree of malignancy often have distant metastasis at an early stage and a poor prognosis. EMT plays an important role [56]. Studies have manifested that inhibition of EMT in osteosarcoma significantly reduces its growth. EMT plays a crucial role in the progression of metastasis of many tumors, and EGF is an important inducer of EMT [57,58]. Liu, et al. [59] found that EGF induces the activation of NF-κB pathway in osteosarcoma cells, and activated NF-κB in turn enhances EGF’s promoting effect on EMT. Inhibition of NF-κB activation with IκBα inhibitor significantly reversed the morphology and viability of osteosarcoma cells, but reduced cell migration. These results suggest that NF-κB plays a key role in EMT of EGF-induced osteosarcoma metastasis. In addition, NF-κB may also promote invasion and metastasis by inducing the expression of transfer-related proteins such as intercellular adhesion molecule-1 (ICAM-1), vascular cell adhesion molecule-1 (VCAM-1), endothelial cell-leukocyte adhesion molecule-1 (ELAM-1) and matrix metalloproteinases (MMPs) [46-50]. These molecules are mediators for cancer cells to migrate, cross blood vessel walls and invade metastatic sites [25,60- 63]. It has been reported that the NF-κB pathway is activated in OS cells in the U20S, and the activation of this pathway up-regulates the expression of metastasis-related proteins Vascular Endothelial Growth Factor (VEGF), matrix metalloproteinase-2 (MMP2), and MMP9, thereby promoting the metastasis of cancer cells. Inhibition of NF-κB activation with QNZ (NF-κB inhibitor) results in downregulated expression of proteins related to metastasis, migration, and invasion, and decreased cell migration and invasion ability [64]. A similar study found that the NF-κB pathway is activated in OS, and activated NF-κB promotes cancer invasion and metastasis by stimulating MMPs secretion [65]. At the same time, Liao, et al. [66] also found that the expression of NF-κB pathway in OS cell line U20S was significantly higher than that in normal bone cells, and the highly expressed NF-κB signal significantly promoted the invasion and metastasis of tumor cells. Using short hairpin RNA knockout NF-κB expression, leading to eliminate the invasion and metastasis of osteosarcoma cells. In addition, a large number of studies have reported that NF-κB pathway activation can promote OS cell invasion and metastasis [67-69]. According to the above studies, it can be determined that activation OF NF-κB is conducive to the invasion and migration of OS, leading to NF-κB may be a new therapeutic target to prevent the aggravation of osteosarcoma.
Promote angiogenesis
Tumor progression is determined by different mechanisms. Most tumors exhibit properties associated with inducing and maintaining angiogenesis, a process that appears to be required for tumor maintenance and progression. NF-κB has been reported to promote angiogenesis and metastasis in some tumor models, possibly by regulating Vascular Endothelial Growth Factor (VEGF) and MMPs [70,71]. Western blot analysis showed high expression of NF-κB in human osteosarcoma cell line SAOS-2, and abnormally high expression of VEGF was detected compared with normal cells. Inhibition of NF-κB activity can reduce the antiangiogenic activity and thus inhibit tumor angiogenesis [50]. In addition, a recent study reported that activation of NF-κB pathway in OS not only promotes tumor cell proliferation and invasion, but also contributes to tumor angiogenesis [47]. Currently, the activation of NF-κB pathway plays a role in angiogenesis after OS, but it has been confirmed that abnormal expression of NF-κB pathway promotes angiogenesis after OS.
Activation of the NF-κB pathway is regulated by a variety of mechanisms and plays a key role in cancer progression including cell growth, invasion, and metastasis. 63 lncrnas have been found to interact with NF-κB pathway to influence tumor progression, such as NKILA, which can be used as a scaffold to recruit multiple proteins in breast cancer metastasis [72]. It also inhibits TGF-β -induced NF-κB activation and acts as a target for breast cancer metastasis by inhibiting EMT [73]. In a study of OS, the researchers learned that the expression of NKILA was significantly reduced in OS tissue, with levels lower than in stage I-II tumor tissue in stage III tumors. Meanwhile, the high expression of NKILA significantly reduced the proliferation, invasion and migration of OS cells. We found that NKILA expression is activated by the NF-κB pathway, which directly blocks the phosphorylation of IκB by interacting with the NF-κB/IκB complex, thereby inhibiting IKK phosphorylation and NF-κB activation. In addition, they found that knocking down NKILA significantly reduced the expression of e-cadherin, which mediates loss of cell adhesion, a prerequisite for tumor cell invasion and metastasis. In addition, NF-κB inhibitors can reverse the effects of NKILA knockdown on cell migration and proliferation [74]. LncRNA X Inactivated Specific Transcripts (XIST) have been found to promote cancer development and be associated with poor prognosis [75-77]. Gao, et al. [78] demonstrated that targeted XIST can activate nuclear NF-κB, P65 translocation and PUMA signaling pathways. Targeting PUMA promoted cell viability and inhibited apoptosis in OS cells transfected with XIST shRNA, suggesting that targeting XIST inhibits cell proliferation and tumogenesis by activating NF-κB and NF-κB dependent PUMA signaling pathways. These findings suggest that XIST may serve as a therapeutic marker for OS therapy.
Due to the importance of the NF-κB pathway in tumorgenesis, a growing number of clinical studies have shown that different natural compounds can inhibit NF-κB activity in cancer cells. Natural preparations or derivatives have always been attractive sources of medicines. Several natural compounds have been shown to inhibit NF-κB. Curcumin, resveratrol and non-steroidal antiinflammatory drugs are currently approved for therapeutic use or undergoing clinical trials. These compounds have shown promise as a treatment in cancer. There is considerable evidence that compounds that block NF-κB activation block the growth of cancer cells [71,79]. Therefore, targeting the NF-κB pathway is a promising treatment for OS.
Curcumin
Curcumin is a natural compound extracted from the rhizome of Curcuma, which has a variety of pharmacological activities such as anti-inflammatory, antioxidant and anti-cancer [80-83]. The anticancer effect of curcumin is related to its ability to induce apoptosis, inhibit inflammation, metastasis and angiogenesis, and sensitize tumor cells to chemotherapy [84-86]. The anticancer effect of curcumin has been proved in many cancers, such as breast cancer, prostate cancer and colon cancer [87-91]. In addition, curcumin has the function of repairing bone defects caused by tumor invasion or surgery [92,93]. Therefore, curcumin inhibits the development of osteosarcoma while also repairing bone abnormalities. Receptor activator of nuclear factor kappa-b ligand (RANKL) is a tumor necrosis factor associated with osteoclast formation, activation and function, as well as increased metastasis rate and cell mobility in osteosarcoma. RANKL is produced by osteoblasts and binds to NF-κB receptor activator (RANK) expressed by giant osteoclasts. RANKL recruited tnFR-associated factors (TRAF; For example, TRAF 1, 2, 3, 5, and 6) lead to the cascade activation of Src/PLCγ, PI3K/Akt/mTOR, and MAPK (P38, JNK, ERK1/2). Transcriptional activators such as NF-κB are activated by TNF-induced IκB kinase (IKK), Fos/Jun, and MITF. Resulting in the transcription of numerous effector genes [94]. Yang, et al. demonstrated that curcumin improves phosphorylation of NF-κB p65 and blocks NF- κB pathway activation [95]. In addition, the cell levels of C-FOS and NFATc1 genes decreased significantly after curcumin treatment. Curcumin has a protective effect on RANKL-mediated osteoclast formation by enhancing macrophage polarization and decreasing activation of NF-кB pathway [95]. Osteoprotegerin (OPG) is a glycoprotein member of the TNF receptor superfamily secreted by osteoblasts. Upregulation of OPG inhibits the interaction between RANK-RANKL and osteolysis. Therefore, down-regulation of RANKL/OPG may play an important role in the treatment of osteosarcoma. Curcumin has been shown to interact with RANKRANKL and reduce its proportion. Therefore, curcumin inhibits the proliferation and invasion of osteosarcoma cells by decreasing the level of RANK-RANKL-OPG [96]. These findings suggest that curcumin can inhibit the progression of OS disease and improve prognosis by inhibiting the activation of NF-κB pathway in OS.
Resveratrol
Resveratrol, a polyphenol phytoantitoxin, is a widely studied phytochemical, and while it is only significantly present in grape products, numerous preclinical studies have investigated its anticancer properties in a plethora of cells and animal models. It has significant pharmacological characteristics and unique ability to regulate multiple targets in different cancers [97]. Resveratrol has been reported to inhibit carcinogenesis by regulating intracellular signaling pathways. The affected signal network controls several key processes, including cell division, growth, apoptosis, inflammation, angiogenesis and metastasis [98]. It has also been reported that resveratrol inhibits cell growth and induces senescence in OS cells (U2-OS) by changing DNA metabolism [99]. In addition, resveratrol can regulate tumor progression by regulating a variety of signaling pathways, including TGFβ1/ SMAD, WNT, SHH/GLI, and NF-κB pathway [100-103]. In some past studies, resveratrol has shown significant effects on NF-κB signaling regulation. Kundu, et al. found that resveratrol inhibited NF-κB activation by inhibiting the phosphorylation and subsequent degradation of IκBα in tPA-treated mouse skin [104]. Res also acts on NF-κB by inhibiting P65 and IκBβ kinases [105]. A recent study reported that resveratrol inhibits NF-κB-mediated cytotoxicity of MG-63 cells in an osteosarcoma mG-63 cell model, thereby inhibiting cell proliferation, migration, and invasion, and activating apoptosis [103]. However, there are relatively few studies on the effect of resveratrol on the pathophysiological progression of OS by regulating NF-κB activation, but it can be confirmed that resveratrol has anti-cancer effects on OS. It may also be a promising multi-target anticancer agent that plays an important role in the prevention and treatment of OS.
Other Natural Inhibitors of NF-κb
Silymarin and silybin (a component of silymarin) form another class of compounds that effectively target the NF-κB pathway. Silybin has been shown to inhibit constitutive activation of NF-κB in DU145 human prostatic adenocarcinoma cells [106]. Parthenolactone is a sesquiterpene lactone, which exists in many medicinal plants and is widely used in folk medicine for its antiinflammatory and analgesic properties. In vitro, it inhibits NF-κB by blocking TNF-α-induced activation of IKKβ [107]. Although there have been no studies showing that these natural inhibitors can inhibit cancer in OS by inhibiting NF-κB activity, these studies suggest that these natural inhibitors may be potential inhibitors to prevent the progression of OS.
As an intracellular signaling pathway, NF-κB signaling pathway is finely regulated, and abnormal expression of this pathway plays a critical role in the development and progression of a variety of malignant tumors, including OS. Structural activation of NF-κB is a novel marker of various tumor types. Many in vitro and animal models suggest that this pathway is involved in multiple steps of cell proliferation, apoptosis, epithelial-mesenchymal transformation, tumor angiogenesis, invasion, and metastasis through complex molecular mechanisms. At present, under the multi-science and multi-mode treatment, the research work on improving the chemotherapy effect has led to the improvement of the survival rate of patients, but the prognosis of OS is not satisfactory, so molecular targeted therapy has gradually attracted wide attention. Combined with the role of NF-κB pathway in OS progression, this pathway may be a potential target for OS treatment and targeting NF-κB pathway may be an innovative approach for the treatment of osteosarcoma as well as a potential target for cancer drug development. It is important to note that NF-κB targeted therapy for cancer (e.g., OS) is a fairly new field that requires optimization in both drug design and patient delivery. Secondly, appropriate delivery systems are required for NF-κB therapy. Therefore, how to select appropriate vectors to ensure successful delivery of NF-κB to the desired target is the direction of future research.
Bio chemistry
University of Texas Medical Branch, USADepartment of Criminal Justice
Liberty University, USADepartment of Psychiatry
University of Kentucky, USADepartment of Medicine
Gally International Biomedical Research & Consulting LLC, USADepartment of Urbanisation and Agricultural
Montreal university, USAOral & Maxillofacial Pathology
New York University, USAGastroenterology and Hepatology
University of Alabama, UKDepartment of Medicine
Universities of Bradford, UKOncology
Circulogene Theranostics, EnglandRadiation Chemistry
National University of Mexico, USAAnalytical Chemistry
Wentworth Institute of Technology, USAMinimally Invasive Surgery
Mercer University school of Medicine, USAPediatric Dentistry
University of Athens , GreeceThe annual scholar awards from Lupine Publishers honor a selected number Read More...