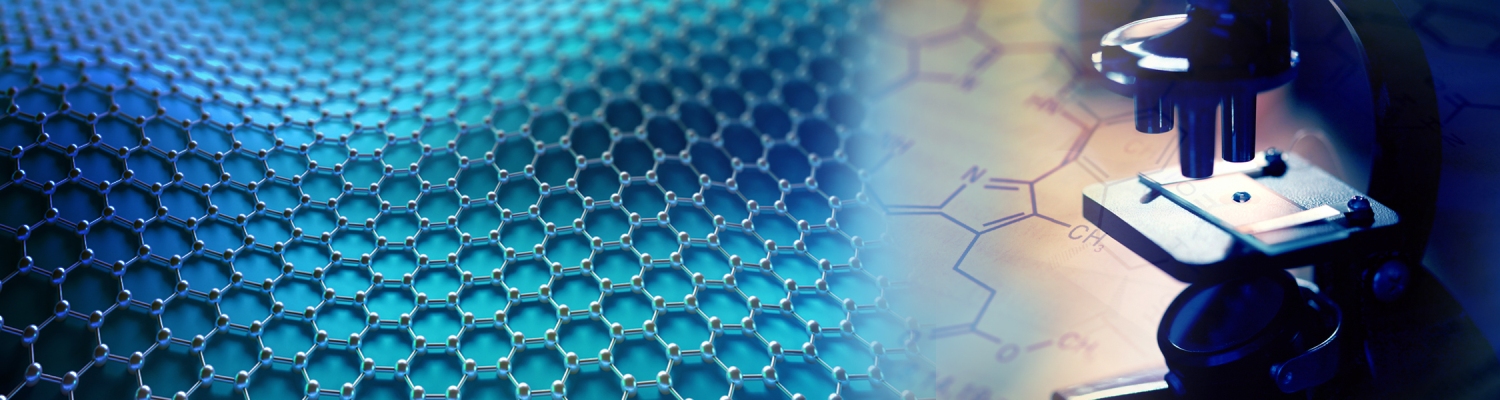
ISSN: 2641-6921
Gunel T Imanova*
Received:June 02, 2021; Published: June 23, 2021
*Corresponding author:Gunel T Imanova, Department of Physical, Mathematical and Technical Sciences, Institute of Radiation Problems, Azerbaijan National Academy of Sciences, AZ 1143 - Baku, Azerbaijan
DOI: 10.32474/MAMS.2021.04.000186
The physical properties and crystal structure of ZrO2 were determined. The basic properties of nanostructured materials are given. The prospects for the use of nanomaterials in the nuclear-power engineering, associated with the creation of nanostructured materials and coatings for structural elements of nuclear-power engineering plant and future thermal nuclear reactor to increase hardness and strength characteristics, raising corrosion and radiation resistance have been considered. The radiation- heterogeneous processes of water decomposition zirconium dioxide (n-ZrO2) nanoparticles have been studied. The kinetics of buildup of molecular hydrogen in the radiolytic processes of water decomposition has been examined. The kinetics of molecular hydrogen accumulation at a gamma radiolysis of water on n-ZrO2 surface is investigated. Influence of gamma radiations on n-ZrO2+H2O systems is studied at various temperatures T=300÷673K. Values of rates of molecular hydrogen accumulation at radiation, radiation-thermal and thermal processes are defined. Deposits of thermal and radiation-thermal processes at accumulation of molecular hydrogen in contact of n-ZrO2 with water are revealed. These results are promising for hydrogen generation by water splitting in near future.
Keywords:Nano zirconium oxide; Radiolysis; Energetic yield of water; Kinetics; γ-radiation; Adsorption
In last years the awareness of climatic change has increasing leading to tempting of exploration of alternative sources of energy. Therefore, the request of green energy is the chief objective for the scientists in 21 centuries. Among various kinds of safe, green, and ecological energy, hydrogen is measured as the best one owing to its low-cost and no toxicity to the environment. The richness of water on the earth is a big benefit for us to generate hydrogen fuel. Hydrogen is created from water-by-water splitting using dissimilar methods. The significant methods include photocatalytic, photoelectrochemical, thermal decomposition and photo-biological radiolysis. Among these, photocatalytic of water is measured as the best one due to green, efficient, inexpensive with comfort of process and with good volume of hydrogen formed [1]. For a long time, dissimilar groups of catalysts are being developed and utilized to split water in the light, but good crop of hydrogen could not be attained at good scale. Nano catalysts have been produced and utilized in water splitting with good achievements. Among these nano zirconium oxides is measured as the best material due to its suitable band gap. Also, the gamma radiation irradiation of the water on the surface of nano catalyst increases the production in hydrogen.
Measurement effects in various compounds with functional properties and their solid solutions, changes in the crystalline dimensions as a result of external influences, and the study of processes such as amorphisationare the main directions of condensed state physics and in organic chemistry [2-7]. As a result of the obtaining and expansion of application capabilities of nano materials, measuring effects become even more relevant. In recent years, the physical and chemical effects of nano-dimensional systems, as well as their unusual properties, have increased interest in the study of these systems and the application of nano oxidesin the field of radiation technology has intensive nature [8- 10]. It is important to search for radiation-resistant materials, to investigate for their various physical properties and to determine the mechanism for changing these properties depending on the radiation dose. Radiation-dependent oxidizations, including Zr and nano-ZrO2, have the most important significance in nuclear reactors [11]. Unlike micro and macro-dimensional oxides, nanoparticle zirconium dioxide compound differs in many ways with its own properties. The main reason for this is completely replacement of the processes in the nanoscale with processes on the surface [12- 14]. Structural studies of zirconium nano particles have shown that monoclinus, tetragonal and cubic phases can be observed in this compound depending on the synthesis method [15]. The structural studies carried out by the neutron diffraction method revealed that the crystalline structure of the ZrO2 compound in the monoclinal phase possesses the spatial group P_1 2_1/c_1 (14) and the lattice parameters are: a = 5.1462 Å, b = 5.2082Å, c = 5.3155Å, β = 99.249° [10]. In the tetragonal phase with higher symmetry, the P_42/nmc (137) spatial group was observed, the lattice parameters were: a = 3.5948 Å, c = 5.1824Å [16]. In the cubic phase, the ZrO2 crystals have the highest symmetry. In the Fm-3m (225) symmetrical cube phase have a = 3.5948Å lattice parameters [17-20]. Due to the diversity of crystal structure and the measurement effect, the physical properties of this compound and the atomic dynamics are also different.The pure zirconia has three different crystal structures: monoclinic, m-ZrO2, tetragonal, t-ZrO2, and cubic, c-ZrO2.
It is known that the characteristic feature of hydrogen production processes in radiation-heterogeneous systems is the conversion of heat and ionizing radiation energy into more efficient forms in solid materials and, finally, hydrogen, which is the main energy carrier in physicochemical processes [10]. The production of H2 in the radiolysis of water absorbed on micron sized particles of CeO2 and ZrO2 has been examined. Radiation chemical yields of H2 increase substantially with decreasing number of absorbed water layers when the yield is determined with respect to the energy deposited directly by γ-rays to the water. These yields reached values of 20 and 150 molecules of H2 per 100 eV for one to two water layers on CeO2 and ZrO2, respectively, as compared to 0.45 molecule/100 eV in bulk liquid water. The yields of H2 determined with respect to the total energy deposited in both the oxide and water were found to have a smaller, but observable, dependence on the amount of water absorbed. Radiolysis of ZrO2 due to γ-rays produced about 5 times more H2 than CeO2 for the equivalent amount of water absorbed. The results suggest that the increase in H2 production is due to the transfer of energy, possibly by an exciton, from the oxide to the water. O2 production was at least an order of magnitude less than H2. The yield of H2 in the 5 MeV helium ion radiolysis of water on CeO2 is the same as with γ-rays, but the results with ZrO2 are substantially lower. The H2 yields with helium ion radiolysis may be nearly independent of the type of oxide.
The formation of H2 in the radiolysis of liquid water containing nanometer sized ZrO2 particles was found to be dependent on the crystalline structure of the particle. Zirconia particles of a few tens of nanometer diameter may be formed with the tetragonal crystalline structure at room temperature rather than the more stable monoclinic form for bulk zirconia. Radiolysis of liquid water containing tetragonal ZrO2 particles exhibits a significant increase in the decomposition of water to H2 as compared to the monoclinic form. Annealing the tetragonal particles to the monoclinic structure results in the loss of excess H2 production above that found with water alone. The results show that surface morphology is extremely important in the decomposition of liquids at solid interfaces, which may have many consequences ranging from nuclear waste storage to the H2 economy. The presented work is devoted to the kinetics and mechanism of formation of hydrogen as a result of the decomposition of water on the surface of nano-ZrO2 [21-23].
Chemicals Used
The nanoscale zirconium dioxide was obtained from SkySpring Nanomaterials, Inc. 2935 Westhollow Dr., Houston, TX 77082, USA. The purity of nanoscale zirconium dioxide was 99.9% with d = 20-30 nm, density ρ = 0.4-0.6 g/cm3 and special surface area S = 330 m2/g. The density and size of nano-ZrO2 are recorded in the passport of sample (by the Company).
Procedures
XRD Analysis: The X-rays were done in the D2-type oscillatory diffractometer (model number-”Bruker D8 Advance”) developed. For this purpose, the dispersed nanoparticles were prepared. This nanoparticular chips was placed in the goniometer of the diffractometer and the X-ray diffraction spectrum of the sample was drawn in the range of diffraction angle 20<2θ<80. Then on the basis of the obtained X-ray diffraction spectra the distance between the atomic flatness (d), intensities of the obtained spectra, syngonia to which the sample belongs, the cage size, density, cage constants and spatial group was determined. The cage parameters are calculated based on the square formulas of crystallography.
Analysis Gases: Analysis of the amount of products (H2, CO2) gases released in the gas phase during the thermal and radiationthermal decomposition of water on the nano-ZrO2+H2O system was carried out by chromatographic method (“Gasochrome-3101”, “Color-102”, chromatographs). The molyar density of gases in 1 ml is calculated by the following formula:
where: molyar density of Ci-i component, molecule/ml or mol/ ml;
height of hi-i-th component, mm;
m - sensitivity of the chromatograph;
Calibration coefficient of the Ki-i component, molecule/mm or mol/mm.
The following formula was used to calculate the substance content of the components obtained in the gas phase during the experiment:
Ni = CiV
where: molyar mass, molecular or mole of Ni-i component;
Molyar density of Ci-i component, molecule/ml or mol/ml;
V- volume of the ampoule tested, ml.
The chromatographic analysis of radiation-catalytic products of water decomposition was carried out. The resulting hydrogen and oxygen products were identified by “Gasochrome-3101” chromatograph. The main conditions of these analyzes are given below. The detector is equipped with two working elements, one for the detection of flammable components by the thermal effect, and the other for the thermal conductivity. It is used to determine non-combustible gases (O2, N, CO2). During heterogeneous radiolysis, water is adsorbed on the surface of nano-ZrO2 and the quality and size of the resulting products are found. Calibration of hydrogen on the chromatograph “Color”-102 and the method of chromatographic analysis of molecular hydrogen formed in the gas phase during the experiment. Calibration of hydrogen in a special calibrator (Gas microdeter № 301) on a chromatograph “Color-102” with a length of 1.0 m and an inner diameter of 3.0 mm of activated (CKT brand) stainless steel column filled with activated carbon (0.25-0.6 mm) conducted. Detector-TCD (detector for thermal conductivity), temperature 50°C, injector temperature 250° C and thermos cabinet temperature were taken as normal room temperature. Ar gas was taken as a gas carrier. The volume velocity of the argon is 60 ml/min, and the operating current of the detector is 80 mA. In SCP-4, the speed of the chart paper was selected to be 600 mm/h. In the studied experiments, the analysis of molecular hydrogen formed in the gas phase was carried out in the same way.
Hydrogen Generation: Analysis of hydrogen and hydrogencontaining gases in a vacuum absorption device was carried out under static conditions. Experimental studies were performed in special quartz ampoules with a volume of V=1 cm3 under static conditions. Sample of 50-70 nm zirconium dioxide were taken as the object of research. The ampoules were filling with the sample of m-ZrO2 =3 x 10-2 g and 0.5 mL water, then were closing in a vacuum absorption device at a temperature of T = 300K until P = 10-2 Pa. In order to prevent oil and lubricants from falling on the samples three nitrogen-cooled holders are connected to the system in vacuum-absorption device. Thermo-vacuum processing of samples is carried out with a zeolite pump at T = 300K, P = 10-3 Pa for 2 hours. The absorption process was the same for both methods (vapor and fluid). The gases generated were inhaled from each adsorbed ampoule to the chromatograph directly.
Radiation-heterogeneous processes were performed at the gamma 60Co isotope source, the dosimetry of the source was determined by ferrosulfate, cyclohexane and methane dosimeters [24]. The irradiation of samples was carried out at the gamma 60Co isotope source. The absorbed dose rate of the source was dDγ/ dt = 0.40 Gy/s [24-26]. The calculation of the absorption dose in these systems was carried out in comparison with the electron density. The absorption dose of gamma quanta was determined in accordance with the methods of ferrosulfate, cyclohexane and methane on the basis of chemical dosimeters in the studied systems.
where: Di - the strength of the absorbed dose in the studied systems;
α -is the ratio of electron densities in the studied and dosimetric systems, which in our experiments varies in the range α = 0.8 ÷ 0.9. Dose - is the strength of the absorbed dose, determined by chemical dosimeters. In the process of thermoradiolysis, the system was irradiated in a special tubular furnace with a temperature of ± 10C. In order to determine the role of radiation processes under the same conditions, the thermal processes of the samples in the studied systems were carried out simultaneously. In order to determine the share of radiation processes in radiation-thermal processes (for example, for hydrogen) can be determined by determining the following formula:
WR (P) = WRT (P) – WT (P)
where: WR(P) - the speed of the radiation process of the product obtained from the raw materials.
WRT(P) – is the of speed radiation - thermal processes.
WT(P) is the speed of thermal processes.
The speed of thermal and radiation-thermal processes is calculated by the following formula:
Here - Nn is the concentration of hydrogen, in molecules / q, τ - radiation time, expressed in minutes.
The radiation-chemical yield of hydrogen G (H2) is calculated by the following formula:
where: - the strength of the absorption dose, expressed in Gr / s.
The total duration of the experiment was 3 minutes.
The pure zirconia has three different crystal structures: monoclinic, m-ZrO2, tetragonal, t-ZrO2, and cubic, c-ZrO2. The crystal structures are shown in (Figure 1) at room temperature, ZrO2 is in a monoclinic phase. Red and blue spheres correspond to oxygen and zirconium atoms, respectively. The phase transition from m-ZrO2 to t-ZrO2 takes place at about 1,447 K. Above 2,650 K, the t-ZrO2 transforms to c-ZrO2, which is stable up to the melting point at 2,950 K. The c-ZrO2 has an ideal fluorite structure (shown in (Figure 1a). The Zr cations are situated in a face-centered cubic (fcc) lattice, i.e. at the corners of the cubic elementary cell as well as at the halves of the [110] directions. The oxygen anions lie at the quarters of [111] directions, which are the tetrahedral interstitial sites asso-ciated with this fcc lattice. In this structure, each Zr cation is coordinated to eight equivalent nearest-neighbor oxygen anions at the corners of a cube, and each O anions is tetrahedrally coordinated to four Zr cations. Consequently, zirconium and oxygen are octahedrally and tetrahedrally coordinated, respectively. The unite cell contains one zirconium and two oxygen atoms with Zr–O distances of 2.2 Å. The t-ZrO2 represents a slightly distorted cubic structure (shown in (Figure 1b), obtained by displacing opposite pairs of oxygen atoms alternatively up and down along the [001] direction. In the tetragonal ZrO2, each Zr cation is still surrounded by eight oxygen anions, with four oxygen-neigh bors arranged in a flattened tetrahedron at a short Zr–O distance, 2.065 Å, and the rest in an elongated tetrahedron rotated 90° at a distance of 2.455 Å from Zr. Each oxygen anion is bonded to two Zr cations at 2.065 Å, and two more Zr cations are 2.455 Å away. The m-ZrO2 has a lower symmetry and a more complex geometric structure, and the primitive cell contains 4 ZrO2, namely 12 atoms (shown in (Figure 1c). There is a large dispersion of inter atomic Zr–O distances, with average values of 2.07 Å and 2.21 Å for Zr–OI and Zr–OII, respectively. (Figure 2) shows the X-ray diffraction spectrum of the nano-ZrO2 compound at room temperature and normal conditions.
Figure 2: X-ray diffraction spectrum of the nano-ZrO2 compound taken at the room temperature and in normal conditions.
Analysis of the X-ray diffraction spectrum by the Rietveld method revealed that the crystal structure of the nano-ZrO2 compound corresponds to the monoclinic symmetry P121/c1 - phase group. The coefficient parameters are: a=5.1481 Å, b= 5.1962 Å, c=5.3132 Å, β=99.25°, Z=4, which corresponds to the results obtained during previous structural studies. The crystal structure of the sample was calculated by the equation
Molecular Hydrogen Generation
The molecular hydrogen occurrence kinetics were investigated by two methods in order to detect the effect on radiolysis of water on the surface of the nano-ZrO2, as well as in the nano-ZrO2+H2O system during radiolytic decomposition of the water, depending on the size of the nanoparticles at T=300K. The speed and energy output rates of hydrogen were determined in the investigated systems based on the initial linear parts of the kinetic curves. It is clear that the kinetic curves of molecular hydrogen formation are almost identical in both systems. In the nano-ZrO2 + H2O system, the yield of G(H2)=2.14 molecule/100eV is ~5 times higher than in pure water [G(H2)=0.45 molecule/100eV]. The surface of oxide compounds is characterized by the predominance of oxygen vacancies. Thus, the surface of oxide compounds is usually positively charged and have an electro-acceptor property. The concentration of surface electro-acceptor centers for different oxide compounds is determined by special probes and is in range L = 1012-1013 sm-2. These charged states usually generate an E = 105-106 V/sm electric field on the surface and affect the different distances depending on the dielectric properties of the environment. Thus, these charged states have a great impact on the migration of charge carriers which form in oxide-type catalysts under the influence of ionizing radiation.
Non-balanced charge carriers can migrate from oxide particles to the surface in two ways.
1. Diffusion,
2. Drift of charge carriers from volume to surface under the influence of a surface electric field (relative distance to the surface)
Charge carriers on the surface level are recombined. As the dispersion is reduced, the yield of localized charge carriers decreases. On the basis of kinetic curves, according to the initial linear regionsthe values of molecular hydrogen formation rate and energy yields were determined and are given in Table 1. Values of radiation-chemical yields are determined by value of rates of the radiation component of radiation-thermal processes of water decomposition. The received values of rates and radiation-chemical yields of molecular hydrogen are given in Table 2. Comparisons of yield values of molecular hydrogen at radiation-heterogeneous processes in ZrO2+H2Oabs. system in the range of temperatures T = 373-673 K, shows that, temperature stimulates process of a heterogeneous radiolysis and the hydrogen yield linearly grows with temperature from 4.8 to 25.7 mol./100 eV. Under the influence of the surface field, the charge carriers migrate from certain depths (λ) on the surfacein accordance with the shown mechanisms. When superficial levels are not present, the particles in that volume are recombined and when surface levels are covered by an electro-water molecule, the carriers are absorbed by them and the water molecules are exposed to radiation-catalytic processes. The presence of water on the surface changes the charge state of the surface. This volume leads to the migration of the holes and the decomposition of water through recombination energy at their superficial levels. If the size of the catalyst used is R, then the following expression is taken for the observed yield of the charge carriers.
Table 1: Estimates of formation rates and energy outputs of the molecular hydrogen during the radiation-heterogeneous radiolysis of water in both cases (n- ZrO2+H2Oabs.and n- ZrO2+H2Oflu..) at temperature T=300K.
Table 2: Values of rates and radiation-chemical yields of molecular hydrogen at radiation-thermal, thermal and radiation processes of water decomposition in n- ZrO2+H2Oabs. system at various temperatures.
Obviously, when λ<< R, Gi = G0, ie, all charge carriers are localized in the volume and the effect of the surface is ignored. When λ = R, then Gi = 0, all formed charge carriers are migrated to the surface and participate in radiation-heterogeneous processes. If the absorption levels of the surface are filled with electroconductive water molecules, the charge carriers are held by them. The effect of surface absorption levels or the effective measurement for radiation-heterogeneous processes is as follows.
Here are: R0 the average dimensions of the nano-ZrO2 particles, Gi-the output when surface levels are water, G0- is the initial release of charge carriers. When Gi = G0, λ = 0, where Gi<< G0, then λ = R0. The migration of energy carriers in radiation-catalytic active oxide compounds varies in the range of 10-103 nm, depending on the composition of the oxides, their structural configuration, and their electro-physical properties. Thus, the effective energy absorption rate in radiation catalytic active acids is limited to these interval dimensions, which in turn leads to the dependence of radiation-catalytic activity on particle sizes, along with other specific parameters. For this purpose, the effect of particle size ZrO2 on radiation-catalytic degradation of water in the range R0 = 20-70 nm was investigated. Radiation-catalytic activity of R0 = 20- 70 nm samples of various modified forms of nanoparticles of ZrO2 has been calculated in the process of water radiolysis. Based on the obtained results, the effective energy transfer distances in the catalysts were determined.
Here, Gi (H2)- replace output with yield molecular hydrogen, G0(H2) - maximum output, G0(H2)=1/2 G0 (non-balanced charge carriers), VcatO. -the specific volume of catalyst (sm3/g), Scat. - catalyst specific surface (m2/g). As it seems, although the particle size is within the micrometer range, effective energy is only at distances measured by nanometers. The effective conversion of the absorbed energy of the ionizing radiation into the hydrogen energy by the catalyst will only be observed in nanoscale samples (λ=R0), with all other parameters stable. The kinetic curve of molecular hydrogen formation during radiolysis of water in the presence of nano-ZrO2 is based on the dependence of hydrogen yield on particle size. The energy output of the hydrogen, based on the obtained results, is 2.14-13.5 molecule/100 eV. This is more than 6 times the maximum value of the radiation-catalytic activity of classic catalysts under the same conditions.
Thus, the energy yield of molecular hydrogen in radiationheterogeneous processes in both nano-ZrO2 + H2Oabs. (H2Oflu.+ nano- ZrO2) systems increases as the particle size of nano-ZrO2 decreases. Such an increase in molecular hydrogen energy yield in comparison with a pure water is explained by the fact that, with the influence of γ-rays, have been formed active centers for water decomposition on the surface of the catalyst at the expense of δ-electrons emitted on the surface of zirconium dioxide nanoparticles. This shows that the dimensions of the studied nanoscale particles are comparable to the free running distance of energy carriers generated by nano-ZrO2 as a result of radiation processes. Thus, radiation-heterogeneous processes in both systems have a measuring effect.
The kinetics of hydrogen formation (a molecular product) via radiation-heterogeneous processes under the influence of gamma-quanta in the system of nano-ZrO2+H2Oabs. is studied. In this process, water molecules are absorbed on the surface of nano- ZrO2 and nano-ZrO2 + H2Oflu.; where ZrO2 suspended (H2O+n-ZrO2) in water. It was found that in a nano-ZrO2+H2O system (nano-ZrO2 suspended in water), the rate of formation of molecular hydrogen [molecule/(g-1·s-1)] and the output of hydrogen (molecule /100eV) per 100eV of absorbed gamma rays was 6.0-6.5 times higher than the ZrO2+H2O system in absorbed condition. This showed when nano- ZrO2 was covered with water, the energy carriers (electrons, holes, excited states-excitons) formed under the influence of gamma-quanta in nano- ZrO2 carried processes of radiolytic decomposition of water molecules both on the surface and in the contact environment. A water + suspension nano-ZrO2 system can be proposed as an effective method for radiolytic decomposition of water in the presence of nano-ZrO2Thus it is established that since T ≥ 473 K there is also an accumulation of the thermal superficial active centers of water decomposition in ZrO2 at thermal-radiation and thermal processes. The monitoring of gamma radiationinduced changes in the surface and the choice of the performance characteristic of a heat-resistant catalytic material based on these nanomaterials. The changes in their physical and chemical properties made it possible to predict the operating modes of the catalytic materials. In this respect, it is of particular interest to study the changes in the surfaces of samples exposed to gamma radiation in comparison with the original samples. It has been established radiation-heterogeneous splitting processes of water with the combined effect of temperature and gamma rays on the surface of nano- ZrO2 goes on electrophysical model with a mechanism.
Bio chemistry
University of Texas Medical Branch, USADepartment of Criminal Justice
Liberty University, USADepartment of Psychiatry
University of Kentucky, USADepartment of Medicine
Gally International Biomedical Research & Consulting LLC, USADepartment of Urbanisation and Agricultural
Montreal university, USAOral & Maxillofacial Pathology
New York University, USAGastroenterology and Hepatology
University of Alabama, UKDepartment of Medicine
Universities of Bradford, UKOncology
Circulogene Theranostics, EnglandRadiation Chemistry
National University of Mexico, USAAnalytical Chemistry
Wentworth Institute of Technology, USAMinimally Invasive Surgery
Mercer University school of Medicine, USAPediatric Dentistry
University of Athens , GreeceThe annual scholar awards from Lupine Publishers honor a selected number Read More...