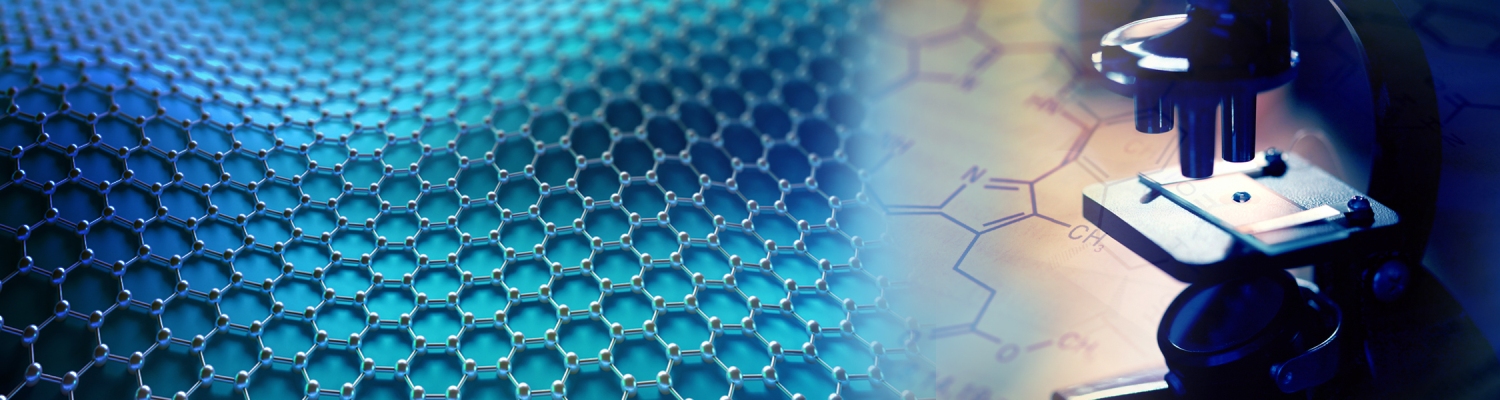
ISSN: 2641-6921
Moslem Mansour Lakouraj1*, Azita Alipour1, Mohsen NajafiRoudbari2 and Reza Ojani2
Received: March 06, 2020; Published: April 08, 2020
*Corresponding author: Moslem Mansour Lakouraj, Polymer Laboratory, Department of Organic Chemistry, Faculty of Chemistry, University of Mazandaran, P.O. Box 47416, Babolsar, Iran
DOI: 10.32474/MAMS.2020.02.000148
A novel biomaterial based on hydrolyzed pectin(HP)/polyaniline(PA)/graphene oxide(GO) has been prepared via chemical polymerization method. The SEM analyses revealed a rod-like and laminated morphology for NH-mGO@HP and NH-mGO@HPPA nanocomposites. Higher thermal stability for NH-mGO@HP-PA was obtained than other samples. Biosensor was designed via electrodeposition of NH-mGO@HP-PA nanocomposite on carbon graphite electrode. Biosensor activity showed a considerable increase in oxidation current by the nanocomposite. The NH-mGO@HP-PA indicated higher electrical conductivity compared to pure PA.
Keywords: Polyaniline; Graphene oxide; Electrochemical properties; Hemoglobin biosensor
Over the recent years, there have been many investigations on
carbon nanomaterials namely graphene oxide due to their high
surface area, electrical and electrochemical properties. GO is a single
sheet of graphite oxide that because of a wide range of functional
groups including oxygen on its surface capable to be modified
chemically with other materials [1-2].GO is recognized as an actual
nanocarbon due to its superior mechanical, structural and thermal
properties relative to other conventional nanomaterials. It can be
synthesized via Hummer method [1,3]. PA/GO nanocomposites
with improved electrochemical performance as energy storage and
supercapacitor were reported in some studies [4-6]. However,there
are few studies on PA/GO nanocomposites as electrocatalyst in
biosensors [7-8]. The poor electrical conductivity of GO caused
by the functional groups creating high electron transfer resistant,
like carboxyl, hydroxyl and epoxy groups in its surface limited its
direct application in electrochemical applications. However, the
incorporation of graphene oxide in a conducting polymer matrix
results in good mechanical strength and electrochemical properties
[9]. PA is one of the conducting polymers which have attracted
much attention in sensor areas as excellent organic conductor
with superior electrical and electrochemical properties, good
chemical and environmental stability and biocompatibility [10].
The combination of PA with other nanomaterials can provide its
application as a desirable matrix for immobilization and detection
of biomolecules via charge transfer reactions [11-14]. Pectin is a
biocompatible high-molecular weight polysaccharide extracted
from cell walls of plants that is composed of three structures of
homogalacturonan, rhamnogalacturonan I and rhamnogalacturonan
II. In spite of its unique properties such as good gelling properties,
biocompatibility, non-toxicity and biodegradability, it has some
inherent flaws that make it unfavorable for application in some
specific fields. The chemical modification of pectin was considered
to remove these drawbacks. Due to the existence of structural
groups such as hydroxyl and carboxyl group on its surface along the
backbone, a wide range of pectin derivatives can be prepared via
chemical modification [15]. In addition, pectin due to possessing functional groups including oxygen can provide a suitable medium
for electron transfer between electrode and electrolyte solution.
In this work we present a nanocomposite hydrogel of NHmGO@
HP-PA for biosensing detection of hemoglobin. In this
work pectin was used as hydrolyzed pectin (HP). The NH-mGO@
HP-PA nanocomposite was synthesized via synthesis of GO with
hummer method, modification of GO via amine treatment with
diethylenetriamine (NH-mGO), immobilization of HP to NH-mGO
by diisocyanate as spacer or coupling agent (NH-mGO@HP) and
finally surface polymerization of PA on NH-mGO@HP to give NHmGO@
HP-PA. The synthesized samples were examined in terms of
structural, morphological and thermal properties via FTIR, XRD,
SEM and TGA analyses. The NH-mGO@HP-PA nanocomposite was
evaluated as biosensor for electrochemical detection of hemoglobin.
This method is a facile, efficient, and green route for development
of a biomaterial based on GO and PA for biological sensors.
Graphite powder, potassium permanganate, sodium nitrate and hydrogen peroxide were provided from commercial source (Merck, Schuchardt Germany). Aniline was obtained from Merck and doubly distilled and purified before use to eliminate any oxidation products. Other chemicals containing diethylenetriamine (DETA), H2SO4 (98%), HCl (37%), NaOH, diisocyanate, acetone and dimethylsolfoxide (DMSO) were acquired from Merck (Schuchardt Germany). Except aniline, other materials were used as received without purification. Ammonium persulfate ((NH4)2S2O8) (APS), hemoglobin (Hb) and glutaraldehyde (GA) were purchased from Asia Pajohesh Company (ltd). Pepsin enzyme was provided from? Pectin was bought from Sigma–Aldrich.
The samples for X-ray diffraction (XRD) analysis were studied by a Philips PW1730 X-ray diffractometer (Netherlands) at a scan rate of 10ºC/min. A FESEM TESCAN MIRA Πdevice (Czech) was utilized to perform scanning electron microscopy (SEM) analysis and to observe the surface morphology of the samples. Fourier transform infrared spectroscopy (FTIR) was used for structural characterization of the samples. The diffraction patterns were recorded using a BRUKER TENSOR27 FTIR spectrometer (Germany), in the range of 400–4000 cm-1in form of KBr pellet. The thermal stability of the samples was attained by thermogravimetric analysis (TGA) with a TGA-DTA METTLER TGA/STTA851 (Switzerland) thermogravimetry under Ar atmosphere at a heating rate of 10°C/min from 25 to 600°C. The electrical conductivity was determined via a four-probe method with the sample prepared as pellet. The electrical conductivity is defined as the below equation:
(1)
in which σ is the conductivity (S cm-1), I is the applied current (mA) through outer probes, V is the voltage drop (mV) measured across the inner probes and d is the thickness of sample (0.1 cm).
The biosensor was fabricated on a carbon graphite electrode (CGE) electrodeposited with NH-mGO@HP-PA nanocomposite and immobilized with pepsin enzyme. A CGE was prepared by graphite powder, paraffin oil, a capillary tube and a copper wire. 160mg of graphite powder was mixed with one drop of paraffin oil. This mixture was mixed in a borosilicate glass vial to form a homogeneous carbon graphite material. A copper wire was inserted into the capillary tube and carbon graphite mixture was manually packed into the capillary to give bare carbon graphite electrode. The obtained CGE was completely polished on a paper. The NH-mGO@HP-PA nanocomposite was electrochemically deposited in an electrochemical cell on three electrodes containing Ag/AgCl as the reference electrode, Pt as counter electrode and carbon graphite electrode as working electrode. After electrodeposition, the electrode was dried at room temperature to obtain a composite modified CGE (NH-mGO@HP-PA/CGE). Afterward, in order to the immobilization of enzyme and detection of Hb, the composite modified CGE (NH-mGO@HP-PA/CGE) was dropped with an enzyme solution containing 4:1 of Hb to pepsin and a GA solution as binder with a ratio of 2:1 pepsin: GA. The electrochemical measurements were performed by a Biological SP150 electrochemical device in an acetate buffer solution containing [Fe(CN)6]3-/4- as electrolyte. The cyclic voltammetry was performed in the range of 500-700 mV at different scan rates and electrodeposition was carried out for 30 min at -600 mV.
Preparation of GO: GO was synthesized by a modified Hummer method according to previous report [1]. In a typical synthesis, a flask containing 3.0g graphite (3.0 g), 1.5g NaNO3 and 69 mL H2SO4 was kept at the temperature below 5°C. Subsequently 9.0 g KMnO4 was added to the flask and the temperature was maintained below 20°C. The reaction was continued for 7h at 35°C. After that, another amount of 9.0g KMnO4 was slowly added to the flask. The reaction system was reacted for 12h at this temperature and cooled to room temperature then diluted by 400 mL of ice water and followed by the slow addition of 3 mL of 30% aq H2O2. The obtained dispersion was washed repeatedly with H2O and HCl to remove the remnant salt. Finally, the resulting precipitate was dried in an oven at 40°C for 24h.
Modification of GO: The amine modification of GO was proceeded via a moderate route by treatment with diethylenetriamine as amine modifying agent according to the prior research [1].A certain amount of GO and diethylenetriamine were loaded in 95% ethanol and sonicated for 40 min. The reaction was performed for 24h at room temperature. At last, the suspension was filtered, washed successively with ethanol, methanol and acetone then dried in an oven at 40°C overnight to give NH-mGO@HP.
Immobilization of HP on NH-mGO@HP: The modification of GO with HP was conducted by a diisocyanate as spacer agent. At first, pectin was hydrolyzed through alkaline method by a NaOH solution (3M) at 50°C for 72h to get HP. The obtained solid product was collected with acetone and dried in an oven at 40°C. To synthesize NH-mGO@HP, the synthesized HP (0.6g) was dispersed in 20 mL DMSO in a 100 mL round-bottom flask equipped with a magnetic stir bar under sonication for 30 min, then diisocyanate (1:10) and 20 % W/W diethylenetriamine as catalyst were added into the above suspension and subsequently was reacted with a dispersion of 0.3g NH-mGO in 20 mL DMSO under nitrogen atmosphere. The reaction was allowed to continue for 24h at 70ºC to twice as high efficiency. Finally, the obtained gelling product was poured into a petri dish and dried in an oven at 40°C.
Preparation of NH-mGO@HP-PA nanocomposite: To synthesize this nanocomposite, a dispersion mixture of NHmGO@ HP in distilled water was prepared via sonication then an aqueous solution of APS (molar ratio of 1:1) was introduced into the above dispersion. After 1h stirring, the polymerization mixture was followed by the addition of an aniline solution with weight ratio of 1:2 (NH-mGO@HP: aniline) in HCl solution (1M). The polymerization was reacted for 24h to be complete. The resultant crude product was filtered, washed with distilled water and acetone and dried in an oven at 40°C overnight. (Figure 1).
The synthetic path of the prepared samples is shown schematically in Figures2-4,(Schemes 1-3).
The FT-IR spectra of the samples of GO, NH-mGO and NH-mGO@HP are exhibited in (Figure2).As exhibited in (Figure2a), the IR spectrum of GO shows representative peaks at ~1009-1168 cm-1, ~1434,1571 cm-1, ~1632,1712 cm-1 and ~3437 cm-1assigned to C-O-C stretching of epoxide rings, C=C stretching of aromatic rings, C=O stretching of carboxyl group, and O-H stretching respectively (1). The infrared absorption spectrum of NH-mGO (Figure2b) reveals new peaks at ~2907-3251 cm-1and also at ~1123 cm-1 ascribed to N-H stretching and C-N stretching vibrations respectively that propose the GO treated with diethylenetriamine via ring-opening reaction of epoxy groups. Furthermore the peaks related to C=O, C-O-C epoxy groups and O-H stretching was decreased in the NH-mGO in comparison with GO that demonstrates the elimination of epoxy functional groups from the surface of GO in the NH-mGO. The modification of the NH-mGO with HP via the reaction with diisocyanate as a spacer agent can result in the creation of urethane and urea functional groups by means of edge carboxyl or surface hydroxyl groups of HP with amine groups of the NH-mGO respectively as can be seen in (Figure2c). The absorption changes happened upon the modification of GO with HP via diisocyanate treatment can be observed via the appearance of the bands at ~1632 cm-1 and ~1693 cm-1 which can be attributed to C=O stretching of urea functional groups and imine NH=C stretching of urethane groups formed respectively. In addition, it is noteworthy that the absence of the peaks associated to the isocyanate groups (2275-2263 cm-1) suggesting the modification of GO and HP with diisocyanate. The main characteristic absorption peaks of PA segments in NH-mGO@HP-PA (Figure2d) were detected at ~1464 cm-1, ~1567 cm-1, ~1288 cm-1 and ~3430 cm-1 asigned to C=C and C=N stretching of benzenoid and quinoid structures, C-N and N-H stretching vibrations respectively.
The surface morphology of the samples was examined by the SEM analyses and the images are illustrated in (Figure3). The micrographs revealed a laminar-like morphology with stacked sheets for GO and nanorod-like morphology for NH-mGO@HP. This change in the morphology can corroborate the stabilization of the HP segments on the GO layers. For the NH-mGO@HP-PA nanocomposite, a typical globular-laminar morphology with embedded GO layers was observed in the SEM image. It can be realized from the micrographs that the coating of the PA on the surface of the NH-mGO@HP nanoparticles reduced the size of the nanoparticles from nanorod to globular shape.
The crystalline structure of the samples was investigated via XRD experiments and the diffractograms are indicated in (Figure4). The XRD pattern of the synthesized GO shows a strong and sharp peak at 2θ=14º corresponding to (001) reflection of GO sheet (9) and peaks at 2θ=24º and 42º corresponding to (002) and (101) reflections respectively. For NH-mGO@ HP and NH-mGO@HPPA nanocomposites, the intensity of the diffraction peaks of GO at 2θ=14º and 42° was reduced. This reduction can be due to the exfoliation of the GO layers with the HP and PA segments. The peak at 2θ=23° appeared with more intensity in these composites compared with GO which illustrates the linkage of HP and PA chains on the surface of GO. Therefore, regarding to the XRD patterns, the results demonstrated the well exfoliation of the GO layers, their desirable linkage to the polymeric composite matrix and a semicrystalline structure for the nanocomposite which may be slightly caused by the presence of the HP segments and also incorporation of GO.
The samples were examined in terms of thermal stability by TGA analysis and the results are shown as curve in (Figure5)and being summarized as comparative data in Table 1. The TGA curve of GO shows a weight loss at 73°C correspond to the removal of residual water. A weight loss occurred at 225°C attributed to the pyrolysis of the labile functional groups containing oxygen and as well the glycoside bonds in GO. The weight loss after 225°C is assigned to the skeletal degradation of the GO. For the sample of the NH-mGO@HP, the temperatures related to the removal of water, scission of the glycoside bonds and also degradation of composite backbone transferred to higher temperatures (168ºC, 339ºC and 449°C respectively) relative to GO. This transfer can be caused by the crosslinking connections existed between the HP segments and GO layers which restrict the free spaces available in the composite and also due to more functional groups containing oxygen that accordingly enhance such these thermal occurrences. The thermal degradation process of NH-mGO@HP-PA happened at higher temperature (at 472°C) in comparison with the NH-mGO@HP.
The scission of glycoside bonds was enhanced to 361ºC. Moreover, a weight loss was observed at 237ºC assigned to the removal of dopant from the nanocomposite. These observations illustrate the influence of the PA chains in the heightening of the thermal stability. It is conceived from the results that with the stabilization of HP on GO in the NH-mGO@HP sample, the thermal stability increased because of the crosslinking linkages between the HP segments and GO layers and also exfoliation and dispersion of the GO layers in the nanocomposite matrix while the residual weight (%) was reduced from 29.45% to 10.2%. This reduction can be attributed to the presence of the glycosidic bonds of the HP molecules in the NH-mGO@HP that accelerates the decomposition of the HP segments and consequently the degradation of the composite. Subsequently the coating of the PA on the NH-mGO@ HP, raised the thermal stability and residual weight (%) of the nanocomposite which is mainly due to the existence of a carbon net structure in the nanocomposite.
The electrical conductivity was measured via four-probe method and results are presented in Table 2. The results show that the presence of GO led to the increase of the conductivity.
The sample was obtained as hydrogel due to high hydrophilicity of pectin. The solubility of the nanohydrogel is shown in (Figure6). As can be observed the biomaterial displayed high solubility in polar dimethylformamide solvent.
The electrochemical biosensing measurement was evaluated via cyclic voltammetry. The cyclic voltammetry responses of bare CGE, nanocomposite modified CGE (NH-mGO@HP-PA/CGE) and the NH-mGO@HP-PA/CGE after dropping of Hb was compared in (Figure7). As can be observed from voltammograms, the bare CGE didn’t show any visible redox peaks while the NH-mGO@HP-PA/ CGE electrode indicated a considerable increase in redox peaks indicating the electrodeposition of NH-mGO@HP-PA nanocomposite on the surface of CGE. This considerable enhancement can be also attributed to the improved electron communication between electrode and electrolyte. After enzyme immobilization and dropping of Hb solution, a well-defined reversible redox peak with a reduction in the redox currents was observed due to the cover of pepsin enzyme to the PANi coating on the surface of electrode. This reduction is indicating the sensitivity of the NHmGO@ HP-PA/CGE to the presence of biomolecule of Hb through the enzymatic detection. Indeed, this observation confirms the biosensing performance of the NH-mGO@HP-PA nanocomposite to detection of Hb. In the cyclic voltammogram of NH-mGO@HP-PA/ CGE, the reduction and oxidation occurred at -0.1 and 0.1 V, which are almost equal, and the redox curves are almost symmetric. The ratio of the redox currents (Ipa/Ipc) was about 1proposing that the electrochemical reaction is reversible. The plausible mechanism of biosensor activity of the nanocomposite is shown in(Figure 8).
A nanohydrogel biomaterial of graphene oxide/pectin/ polyaniline was synthesized. Thermal stability and electrical conductivity were increased in the NH-mGO@HP-PA nanocomposite. Electrodeposition of the nanocomposite enhanced the cyclic voltammetry. Biosensor showed the electrocatalytic activity of nanocomposite to detection of hemoglobin.
In the present work a nano biomaterial based on HP, PA and GO was synthesized via chemical modification and polymerization. The samples were characterized via FTIR, XRD, SEM, and TGA/ DTA analyses. The nanocomposite showed an enhanced electrical conductivity relative to pure PA. The SEM images showed a nano spherical/laminate morphology for nano biomaterial. The TGA/ DTA analysis indicated higher thermal stability for the NH-mGO@ HP-PA nanocomposite compared to pure GO. The biomaterial was studied in terms of biosensing application for detection of hemoglobin. The NH-mGO@HP-PA/CGE electrode showed an enhanced cyclic voltammetry in the presence of the nanocomposite due to the increase of electron transfer and a reduction in the redox currents due to the cover of enzyme to the PANi coating on the surface of electrode which indicating the sensitivity of the NHmGO@ HP-PA/CGE to hemoglobin detection.
We appreciate the deputy of finance of university for partial supporting this work.
There are no conflict of interest to declare.
Bio chemistry
University of Texas Medical Branch, USADepartment of Criminal Justice
Liberty University, USADepartment of Psychiatry
University of Kentucky, USADepartment of Medicine
Gally International Biomedical Research & Consulting LLC, USADepartment of Urbanisation and Agricultural
Montreal university, USAOral & Maxillofacial Pathology
New York University, USAGastroenterology and Hepatology
University of Alabama, UKDepartment of Medicine
Universities of Bradford, UKOncology
Circulogene Theranostics, EnglandRadiation Chemistry
National University of Mexico, USAAnalytical Chemistry
Wentworth Institute of Technology, USAMinimally Invasive Surgery
Mercer University school of Medicine, USAPediatric Dentistry
University of Athens , GreeceThe annual scholar awards from Lupine Publishers honor a selected number Read More...