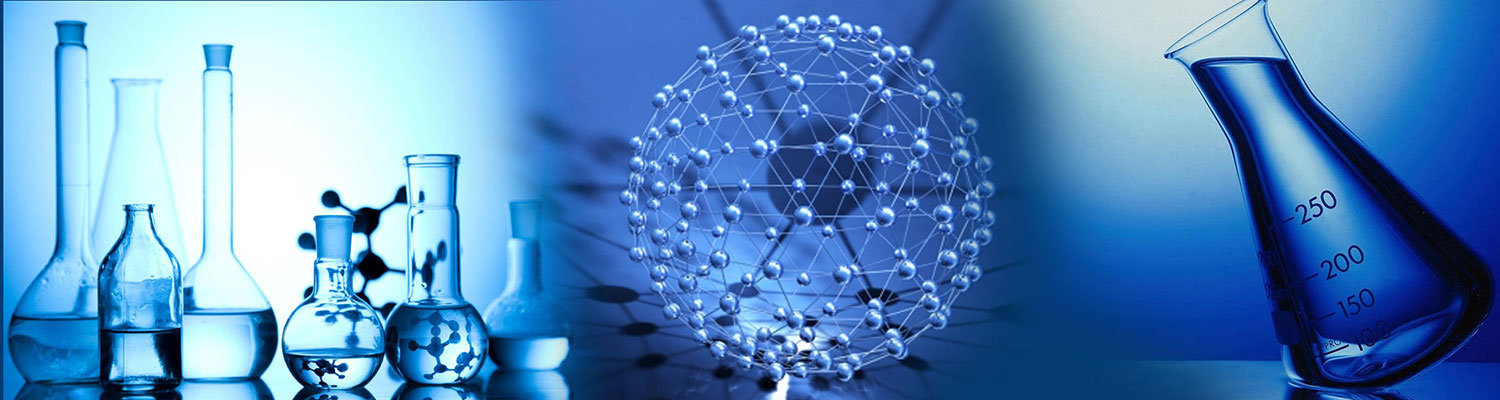
ISSN: 2637-4609
Xiang Zhangb2, Yang Shi1, Shiguo Xua1*, Xiaoyan Zhoua1, Kaihua Xub2*, Yujun Zhanga1*, Wei Lia1*, Ningjing Liao1, Haibo wangcde3,4,5, Jianqing Zhao3,4
Received: June 27, 2020; Published: August 10, 2020
*Corresponding author: Shiguo Xua,GEM new energy materials research institute, GEM (Wuxi) energy material CO., LTD, Wuxi, 214142, China
DOI: 10.32474/AOICS.2020.04.000193
Li(Ni0.82Co0.11Mn0.05Al0.01)O2 (NCMA) cathodes are synthesized by adding Al2O3 during high temperature solid state reaction. The results show that Al element can largely increase the surface stability of cathode compared to pristine NCM cathode and thus suppress the severe capacity fading after washing process. NCMA delivers a discharge capacity of 203.8 mAh g-1 at 0.2 C, with an outstanding capacity retention of 94% after 50 cycles at 25°C. This proposed synthesis strategy demonstrates that an optimal doping method will immensely retain the electrochemical performance while reduce content of the residual lithium compounds during the washing process which promote industrial fabrication of cathode materials.
Keywords: NCMA; washing process; cathode material; Li-ion battery Abbreviations: Li(Ni0.82Co0.11Mn0.05Al0.01)O2 (NCMA); Li(Ni0.83Co0.12Mn0.05)O2 (NCM); Scanning electron microscope (SEM); X-ray diffraction spectra (XRD); Cyclic voltammetry (CV); Electrochemical impedance spectroscopy (EIS)
Lithium-ion batteries (LIBs) have been widely studied to meet the growing demand for the portable electronic devices and electrical vehicles [1, 2]. The LiNixCoyMnzO2 cathode materials (NCM) are considered to be the most promising cathode materials due to the high specific capacity and low capital cost [3, 4].Notably, the specific capacity of NCM can be improved by increasing the Ni composition in the layered structure. However, the Ni-rich cathodes with nickel content above 80% suffer from poor surface stability which hindered the practical application of the Ni-rich cathode materials [5-8].
Compared to NCM523, NCM811 is more susceptible to atmosphere as NCM811 is a fairly hygroscopic material [9]. The residual lithium compounds such as LiOH and Li2CO3 originate from spontaneous surface reduction with moisture and air are strongly related to the safety issue and battery performance [10-14]. Li2CO3 is responsible for the gas generation which may be arise from the decomposition reaction with electrolytes when charged to high voltage (>4.1V) in the cell [15-17]. On the other hand, LiOH will increase the pH value thus causing the gelation of the slurry during the electrode fabrication process [3]. Hence, the washing process was adapted to remove the residual lithium compounds of Ni-rich cathode. Although washing process greatly reduce the residual lithium compounds and pH value, the direct contact with water will facilitates the phase transition from the layered structure to the NiO like structure which shows no electrochemical activity [18]. The degradation of surface is fatal to the electrochemical performance and practical application of Ni-rich cathodes [19-21].
Recently, Kim et al. report the quaternary layered Ni-Rich cathode which maintain the specific capacity and excellent cycling stability [22, 23]. In addition to the suppression effect of Al-doping during the H2-H3 phase transition, the substitution of Al element greatly reinforces the surface of cathode. However, quaternary layered Ni-Rich cathode still suffer from residual lithium compounds and few paper shows the battery performance of the NCMA cathode materials before and after washing process. In this paper, we report on the structure and electrochemical properties of NCMA and NCM before and after washing process by SEM, XRD, CV, EIS and galvanostatic cycle analysis.
The NCMA quaternary layered materials were synthesized by the solid-state method using LiOH (Ganfeng Lithium Co. Ltd), transition-mental hydroxide precursors Ni0.83Co0.12Mn0.05(OH)2 (GEM Co. Ltd.) as raw materials and a trance amount of Al2O3 (Sigma) was added as doping additive. The Ni0.83Co0.12Mn0.05(OH)2 precursor was mixed thoroughly with LiOH (Li:Ni+Co+Mn=1.02:1) and Al2O3 and then the mixture was calcined at 800℃ for 12h in oxygen. For comparison, the NCM cathode materials were synthesized by mixing LiOH and Ni0.83Co0.12Mn0.05(OH)2 precursor (Li:Ni+Co+Mn=1.02:1) and then calcined at 800℃ for12 h in oxygen.
The positive electrode material after sintering and crushing was mixed with purified water in a mass ratio of 1:1 and then stirred for 5 min. The materials were collected through filtering and finally dried at 120℃ in a vacuum oven, which were recorded as NCMAWD and NCM-WD, respectively.
The surface morphologies of the cathode materials were observed with the FEG250 scanning electron microscope produced by FEI Company. The test voltage was 5-15kv and the working distance was 10-11 mm. The phase and crystal structure of the materials were characterized by the XRD-7000 X ray diffractometer produced by Japan shimon using Cu-Ka radiation at 40kV. The scanning speed was 2°/min, and the 2 theta Angle was 15-75°.
The X-ray Rietveld refinement was performed by the General Structure Analysis Software (GSAS) package with the EXPGUI interface. The refining parameters included background coefficients, lattice parameters, peak shape parameters, the positional parameter of O (6c), the fractional factors of all Li, Ni, Co, Mn and Al [24-26].
Electrochemical properties of the cathode materials were evaluated using CR2032 coin-type half-cells. The prepared cathode materials were mixed with conductive carbon and polyvinylidene fluoride with a mass ratio of 80:10:10. Then all above materials were dissolved in N-methyl pyrrolidone solvent (NMP) and mixed uniformly using a ball mill. After mixing evenly, the uniform slurries were coated on the aluminum foil and dried at 120℃. Then the coated aluminum foil was punched into pellets with a diameter of 13 mm and dried in a vacuum oven for 8 h. Then the CR2032 coin-type half-cells were assembled in the order of battery shell (buttom), negative plate (lithium wafer), diaphragm, electrolyte, positive plate, reed, and battery shell (up) in a glovebox under argon atmosphere. The active mass loading was about 9 mg/cm2. The electrolyte was 1.0 M LiPF6 dissolved in a mixture of ethylene carbonate (EC), dimethyl carbonate (DMC), ethyl methyl carbonate (EMC) with the volume ratio of 1:1:1. The Discharge-charge tests were performed at the charge or discharge ratio of 0.5C on a LAND battery test system produced by Wuhan blue electric company. Cyclic voltammetry and AC impedance tests were carried out by the electrochemical workstation Solartron (1287+1260) with the scanning speed of 0.1 mV/s and voltage range from 3.1 V to 4.5 V for cyclic voltammograms test. The frequency range of AC impedance test was 0.01 Hz–105 Hz, and AC amplitude was 100 mV.
Figure 1 shows the SEM images of pristine and washed powders
of NCM and NCMA cathode materials. All of the powders display
a spherical morphology and each spherical secondary particle is
composed of an agglomerate of primary particles. The exquisite
observation on the NCMA surface shows that edges and corners of
particles became obscure and the primary particle size distributes
larger compared to the pristine NCM which indicates more interact
between the primary particles. In addition, the primary particles
were found removed during the washing process as is marked in
Figure 1c while no obvious defects were detected in NCMA powders.
This implies that introducing of Al element into the NCM materials
improves the spatially correlation of primary particles.
Figure 2 shows the X-ray powder diffraction patterns of the
samples. All of the samples exhibit a well-defined layer structure
based on a hexagonal α-NaFeO2 structure with a R-3m space group
without any impurity phases [27]. The clear peak splits of the
(006)/(102) and (018)/(110) peaks are observed for all samples,
indicating that all the samples have well-defined layered structures
[28]. Rietveld refinement results shows that the Al element decrease
the Li/Ni exchange from 1.9% (NCM) to 1.6% (NCMA).
Figure 3 shows the cyclic voltammetry analysis of all materials between 3.1 and 4.5 V (vs. Li/Li+) at a scan rate of 0.1 mV s-1 in the first cycle. It can be seen that the four materials have similar redox peaks at 4.16V/4.22V, which corresponds to the platform near 4.20V on the charge-discharge curve and represents the transformation between H2 and H3 of the hexagonal crystal phase. The redox peak at 3.71V/4.06V corresponds to the redox process of Ni2+/Ni4+ [29-31]. Tiny cathodic peaks P0 and P4 are observed at around 3.50V and then disappear in the subsequent oxidation process, which may be attributed to the irreversible decomposition of impurities at the electrode/electrolyte interface as no relative cathodic peaks are detected in the washed samples. As shown in the cyclic voltammetry curves, the overpotential between first redox peaks (ΔV) of NCM and NCMA are 0.350 V and 0.345 V, while the ΔV of NCM-WD and NCMA-WD are 0.353 V and 0.323V, respectively. The washing process decrease ΔV of NCMA-WD by 0.02 V, which indicates that the washing process will remove the residual lithium compounds on the material and increase the reversibility and reactivity of the cathode material. On the other hand, NCMWD shows larger overpotential than NCMA-WD which should be attributed to the stable effect of Al doping on the structure. CV curves present that the Al doping modify the surface of the powders and suppress the destruction of water.
Figure 4 shows the initial charge-discharge curves for the four materials tested at 0.2 C. There is no significant difference between the initial discharge capacities of NCM and NCMA of approximately 192 mAh g-1. However, NCM-WD and NCMA-WD show larger initial discharge capacity of 200.4 and 203.8 mAh g-1 which may be arise from the removing of impurity on the surface by washing process.
Figure 5 shows the cycle performances of all the materials, NCMA shows similar capacity retention with NCM while NCMA-WD shows greatly improved capacity retention compared with NCMWD. It should be noted that after washing process, both NCM-WD and NCMA-WD show superior capacity which may be arise from the remove of residual lithium compounds but the poor capacity retention of NCM-WD is derived from the structural instability of the material after washing process.
EIS spectra of samples has been measured after 1 cycle and 50 cycles as shown in Figure 6. The plots consist of two semicircles in the high frequency region. The semicircle at high frequency could be attributed to the resistance for Li ion migration through surface film (RSEI) and semicircle at high-to-medium frequency is assigned to the surface charge transfer process (Rct). The cathodes show similar surface film resistances, but the charge-transfer resistance, Rct, differed substantially depending on the treating process. The Rct value increased appreciably from the surface decomposition during washing process. Al element dramatically decrease the Rct which should be assigned to the surface stabilization of the Al element. In addition, the value for Rct of NCM gradually accumulated after 50 cycling and obviously vary from the NCMA although they have similar Rct after 1 cycle. As shown in Figure 5, NCMA-WD maintain the excellent ability of Li conduction after washing process while NCM-WD after 50 cycles shows tremendous Rct value compared with that of the pristine material for the structural degradation of surface during washing process.
We synthesized NCMA cathodes by high-temperature solid state method. The partial substitution of Ni with Al element can facilitate the growth of primary particles and improve the spatially correlation of primary particles which reduce the destruction of secondary particles during washing process. Optimal Al-doping reduce the overpotential of the cathode and retain the capacity of the material. Moreover, the EIS analysis shows that the Al-doping can retard structural degradation of surface and maintain the capacity retention during washing process. The NCMA cathode after washing process delivers a discharge capacity of 203.8 mAh g-1 at 0.2 C with an outstanding capacity retention of 94% after 50 cycles at 25°C. This proposed synthesis strategy demonstrates that an optimal doping method will immensely retain the electrochemical performance while reduce content of the residual lithium compounds during the washing process which promote industrial fabrication of cathode materials.
The authors declare no competing financial interest.
This work was supported by Industrialization Project of Wuxi (WX18IVHC719).
Bio chemistry
University of Texas Medical Branch, USADepartment of Criminal Justice
Liberty University, USADepartment of Psychiatry
University of Kentucky, USADepartment of Medicine
Gally International Biomedical Research & Consulting LLC, USADepartment of Urbanisation and Agricultural
Montreal university, USAOral & Maxillofacial Pathology
New York University, USAGastroenterology and Hepatology
University of Alabama, UKDepartment of Medicine
Universities of Bradford, UKOncology
Circulogene Theranostics, EnglandRadiation Chemistry
National University of Mexico, USAAnalytical Chemistry
Wentworth Institute of Technology, USAMinimally Invasive Surgery
Mercer University school of Medicine, USAPediatric Dentistry
University of Athens , GreeceThe annual scholar awards from Lupine Publishers honor a selected number Read More...