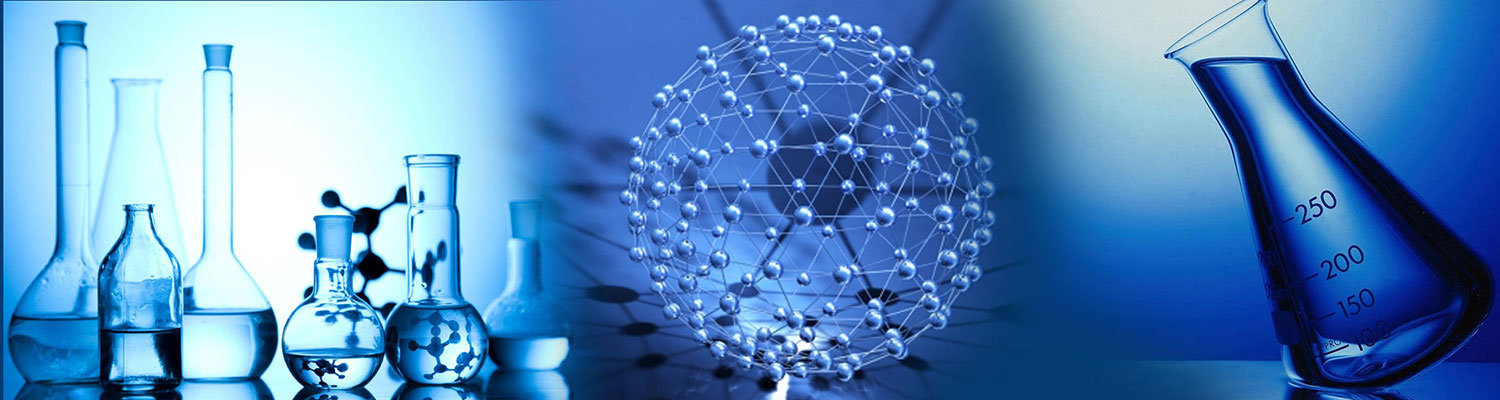
ISSN: 2637-4609
Drochss P Valencia1, Gerardo Cebriàn-Torrejòn2*
Received:April 1, 2021; Published:April 20, 2021
*Corresponding author:Gerardo Cebriàn-Torrejòn, Laboratories COVACHIM-M2E (EA 3592), University des Antilles, France
DOI: 10.32474/AOICS.2021.05.000205
Since the first developments of electrochemical sensors for the determination of biological molecules of interest, a great variety of electrochemical aptamer-based devices have appeared to detect innumerous biomolecules of interest [1-6]. Most of these developments are like blood glucose meters (scheme 1) [1] and with them a fast sensitive, low-cost and easy-to-operate detection is achieved [1]. These devices are known as biosensors because they use a transducer signal, making it possible to correlate the electrochemical reactions that occur on a surface called an electrode with a quantifiable signal [1, 7]. These systems allow researchers to provide selective analyzes in complex matrix (e.g., blood, saliva, urine, among others) [1-6] unlike other analysis methods (e.g., optical, mechanical, or mass coupled chromatographic methods [1-5]. Electrochemistry response (current or potential) can be a proportional function of the species concentration and the time of applied stimulus (potential or current) respectively. Electrochemical analysis techniques are distinguished through the following experiments: Electron transfer processes (differential pulse voltammetry (DPV) [4-9], chronoamperometry (CA) [2], cyclic voltametry (CV) [4-8], square wave voltametry (SWV) [4, 7]), and load transfer resistance processes (electrochemical impedance spectroscopy (EIS) [4-8]). In addition, the modification of the electrodes allows the specific recognition of molecules of biological interest, adding specificity in the analyses [2-8], which is required in a complex biological matrix. In this sense, electrochemical biosensors have become the perfect tool for the direct detection of biomarkers [2, 4, 5], and their current developments towards the determination of diseases such as severe acute respiratory syndrome coronavirus 2 (Sars cov 2).
The electrochemical biosensors present several benefits for, such as low reagent/sample volumes [1-10], short analysis times [1-10], feasible sample treatments [1-10], among others [1-10]. These favorable characteristics are achieved by optimizing the modification of the sensing materials and the immobilization methods [2-10]. Also, modifying agents may contain different redox properties from the analyte to be studied, which ends up giving the biosensor the high specificity sought in an analysis [2, 6]. The development of nanotechnology also ended up playing a decisive role in the development of electrochemical biosensors [6, 10]. Nanoparticles [6, 11], quantum dots [6], graphene [6- 12] and nanotubes among others [6, 12] have been used to performance the efficiency of electron/charge transfer processes and to increase the active surface area [2-10]. As consequence of this, the quantifiable signals increase and the detection limits decrease [2]. The development of nanotechnology has contributed to the miniaturization of instrumentation and the portability of biosensors [1,10]. This flexibility allows the projection and development of equipment that can be used for the determination of COVID-19 with a smartphone [10].
Once of the factors that has contributed to the severity of the pandemic was the lack of rapid diagnosis methods and studies on the infection progress [6, 9]. The problem with the tests that involve the genetic analysis of the virus, are the diagnosis times, and the detection limits only being measurable after the third day of the disease. The tests to detect SARS-CoV-2 include the identification of genetic material of the virus, viral antigens, or tests serological antibodies. The present review we will explore some of the electrochemical developments that show improvements in the diagnosis of the disease with biosensors. Also, the development of equipment for determining the disease will be discussed.
Electrochemical Geno sensors
The biosensors that use molecular recognition elements for DNA and RNA are known as Geno sensors [3-13]. For the construction of these, the surfaces of the electrodes are modified with sequences paired to the DNA and RNA to be detected [3]. If the analyte of interest is present in a studied matrix, the response of the electrochemical Geno sensor changes. This change is quantifiable and proportional to the concentration of the analyte, indicating the presence and/or absence of the molecule of interest [3-13]. In general, the efficiency of these Geno sensors has been demonstrated, comparing their responses with biochemical techniques (such as RT-PCR (reverse transcription polymerase chain reaction) [6, 14], antigen or antibody detection by ELISA assay [6, 9], hemagglutination assay [6], nucleic acid detection and gene sequencing [6]). Most of the time, the Geno sensors present sensitivities comparable or better that these procedures. Their sensitivities can be optimized and improved through different techniques for treating the sample, modifying the surface, the type, and shape of the electrochemical technique developed for the determination of the analyte of interest [15].
Different Geno sensors have been developed, for example, for the determination of Factor V Leiden Mutation [3], hepatitis B virus [6, 8], human papillomavirus (HPV) [2], human immunodeficiency virus (HIV) [6], breast-cancer BRCA1 gene [7], avian influenza [4] pathogenic rotavirus [9] among other diseases. All these Geno sensors are based on the chemical and physical modification of surfaces such as carbon [3-7], gold [3], platinum, palladium among others, with chemical species of biological origin, such as portions of DNA [3], RNA [7], modified peptides. Their detection limits include scales from 10-6 to 10-15M [3,7], which demonstrates its high sensitivity for the determination of viruses and in early stages of the different diseases that are presented by them. Based on these findings, developments of Geno sensors for the determination of SARS COV 2 have been carried out. The biochemical analysis allows to establish 4 main structural proteins (protein (S), protein (M), protein (E) and protein (N)) and the genes of the accessory proteins (protein (HE), 3, 7a, among others) [16]. The identification of these proteins by Geno sensors allows us to detect the infection in early stages. Most of biosensors have chosen the determination of the protein S because the different mutations of the virus only produce few changes in. Also, its concentration is higher with respect to the others [16]. To develop a Geno sensor and determine SARS COV 2, portions of the target genome, were immobilized on different types of surfaces. For these developments different types of gold surfaces are covalently modified and hybridized with the genetic material of the virus. The first studies included the immobilization of some complementary pyramidic bases of the virus genome on gold surfaces. The immobilization can be achieved by sputtering or by depositing nano-structured gold on printed graphite electrodes [16]. The modification of these surfaces is complemented with a biotinylating, followed by surface hybridization processes [16]. These two developments permit to reach detection limits (LOD) of the order of 2.5 to 6.0 Pm. These procedures decrease the time for the determination of the disease compared with other techniques such as RT-PCR [3] (from 1 to 4 hours), antigens and antibodies (from 1 to 5 hours) with LOD of the order of 0.4 pg/mL. In addition to requiring specialized laboratories for these determinations. As can be seen with these first works, electrochemical techniques allow much faster analysis and permit to use lower sample volumes.
Electrochemical Geno sensors based on bioinorganic complex
Other Geno sensors were developed using bioinorganic complexes in solution, such as the Au (I) complex, sodium aurothiomalate or co-porphyrins [4]. These electrochemical tags allow determining the presence of the virus by indirect measurements. These Geno sensors are faster than the ones previously described (detection the time was decreased to the half), but the sensitivity was not so high (with LOD of the order of 0.5 nM up to 8 pM). Another similar approach, employ a typical redox probe in electrochemistry ([Fe (CN)6]3–/4–), to determine or not the presence of the virus due to changes in the electrochemical response by differential pulse voltammetry (DPV). By this technique, the determination of the influenza virus in 1 pM was reported. Improvements in the development of the Geno sensor implied changes in the starting metal surface, where it is replaced by carbon surfaces, which are modified in the form of avidin/biotinylated surface [7, 13], and reaching detection limits of the order of 85.1 fem. By these last work surfaces avoided the use of redox mediators for the determination of the influenza virus [4], which facilitates the development of devices at the research laboratory level.
Electrochemical Antibody based sensors
A different strategy is based on the use of antibodies to reach the molecular recognition of the virus. Antibodies are placed on surface and allow the change of a signal in the presence or absence of the virus. Some authors have used graphene sheets immobilized on vitreous carbon electrodes and modified with antibodies against specific proteins of the virus. For example, immobilization has been carried out by coupling the virus’s proprietary antibodies with N-hydroxy succinimide and 1-pyrene-butyric acid on the graphene sheet. The nature of these antibodies on the electrode surface allowed the construction of a highly sensitive and selective SARSCoV- 2 biosensor. The sensor has an analysis range of 1 fg/mL to 100 fg/mL for the determination of SARS-CoV-2 spike protein in clinical transport electrolyte solution. The biosensor also allowed the detection of 16 plate forming units per sample unit volume (PFU/ mL) in culture medium, as well as in clinical samples, patients with at least 250 copies of the virus per mL can be determined without any preprocessing or preparation.
Impedance based sensors
Importantly the lack of electrochemical activity of the coronaviruses, made most of the developments of Geno sensors for SARS COV 2, were considered as electrochemical impedance studies. The transduction signal is an imaginary impedance signal as a function of the real impedance. A signal that allows to simulate an electrical circuit that has physical correspondence with the elements of the electrode. As consequence the changes in the resistance of the surface can be attribute or not to a positive diagnosis of a patient. These electrochemical impedance studies have been performed successfully for different types of influenza viruses, including IAV, H1N1, H5N1 [4], and H7N9 [6].
Thanks are due to the AUF (Agence Universitaire de la Francophonie, COVIDCHIM project) and French Research Department (DICHLOAL-MAREC projet) for the financial support to GCT. DPV Acknowledges to COLCIENCIAS by the OMICAS program: Optimizacion Multiescala In-silico de Cultivos Agrıcolas Sostenibles (Infraestructura y validacion en Arroz y Caña de Azucar), sponsored within the Colombian Scientific Ecosystemby The WORLD BANK, COLCIENCIAS, ICETEX, the Colombian Ministry of Education and the Colombian Ministry of Industry and Tourism under GRANT ID: FP 44842-217-2018
Bio chemistry
University of Texas Medical Branch, USADepartment of Criminal Justice
Liberty University, USADepartment of Psychiatry
University of Kentucky, USADepartment of Medicine
Gally International Biomedical Research & Consulting LLC, USADepartment of Urbanisation and Agricultural
Montreal university, USAOral & Maxillofacial Pathology
New York University, USAGastroenterology and Hepatology
University of Alabama, UKDepartment of Medicine
Universities of Bradford, UKOncology
Circulogene Theranostics, EnglandRadiation Chemistry
National University of Mexico, USAAnalytical Chemistry
Wentworth Institute of Technology, USAMinimally Invasive Surgery
Mercer University school of Medicine, USAPediatric Dentistry
University of Athens , GreeceThe annual scholar awards from Lupine Publishers honor a selected number Read More...