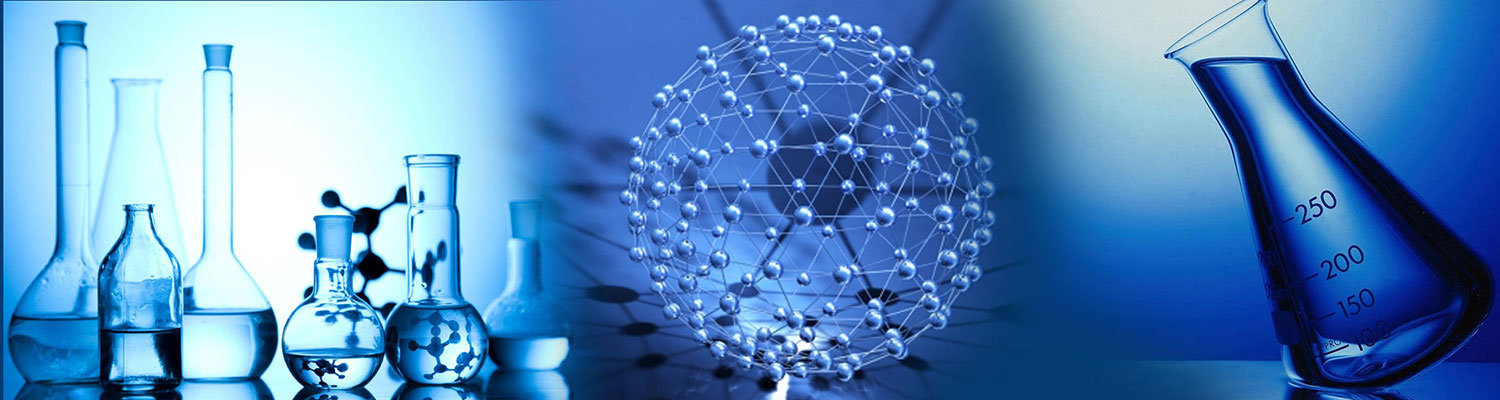
ISSN: 2637-4609
Kaana Asemave*1 and Andrew J Hunt2
Received: May 16, 2018; Published: May 18, 2018
*Corresponding author: Kaana Asemave, Benue State University, Nigeria
This research demonstrated the purification of a lipophilic β-diketone (14,16-hentriacontanedione) from wheat straw wax using greener alternative organic solvents; cyclohexane, p-cymene, 2,2,5,5-tetramethylTHF (TMTHF) and cyclopentyl methyl ether (CPME); and then supercritical carbon dioxide (scCO2). The purification of the 14,16-hentriacontanedione (Htd) with cyclohexane, TMTHF, CPME and p-cyme involves crashing the lipophilic β-diketone from the wax with cuprous acetate. The results of the purification of Htd using these greener alternative organic solvents showed that TMTHF gave the highest yield of Htd as 21.40wt%, while 6.0 wt% yield of Htd was found with cyclohexane. However, cyclohexane, TMTHF or CPME can be effectively used as greener alternative for the isolation of Htd. Again, sequential supercritical carbon dioxide (scCO2) fractionation of the wax was carried out in order to obtain Htd using different adsorbents (celite, silica and alumina) and varying pressures. The sequential scCO2 fractionation of 1g wax: 200g silica at 200 bar and 300 bar at 313 K for 40 min was found to give best selectivity for Htd than with alumina and celite adsorbents under the same condition.
Beta-diketones are very important type of compound with many applications. They have been used as ligands for over 120 years [1,2] as well as NMR shift reagents, laser chelates, metals chelating agent, chemical and photochemical catalyst, antitumor, antioxidant, anti-inflammatory and antiviral agents. [1,3]. For instance, lipophilic biobased β-diketone (14,16-hentriacontanedione) has been reported as greener alternative metal chelating agent [4,5]. Though the traditional chelators (aminopolycarboxylates and phosphonates) have strong chelation effects for metals [6,7], however, it has been found that most of these compounds are not readily biodegradable [6,8,9]. The infiltration of these chelants into the environment causes dissolution of heavy metals from the sediments and soils, thereby mobilizing them [10-13] thus leading to increased levels of metals [14]; accept phosphonates that do not mobilise toxic metals [9,11]. Hence, it has become necessary to look out for greener alternatives for chelators.
Moreover, more than 90% of organic chemicals are derived from fossil fuel refineries [15,16] which is not sustainable. Hence there is high attention and desire to source for bio based chemi cals for the sake of sustainability and to a large extent safety. Wheat straw is an abundant and low value bio-feedstock in many countries, especially in Europe [17]. Del Río [18] reported that lipid quantity in the wheat straw constitutes 1-2%. Wheat straw wax has been highlighted as a potential renewable wax with many attractive characteristics to replace some existing commercial waxes in cosmetic applications [19]. The wax offers protection against particle accumulation of pathogens by repelling water on the surface of the plant [20]. Furthermore, it prevents the formation of water films on the surface, which reduces gas exchange dramatically [20]. Studies have shown that the lipophilic content of wheat straw wax comprisesn- fatty acids (25%), n-fatty alcohols (20%), n-alkanes (5%), n-aldehydes (1%), high molecular weight esters (11%), monoglycerides (2%), diglycerides (1%), triglycerides (2%), β-diketones (10%), steroid hydrocarbons (<1%) and steroid ketones (1%) [18]. Furthermore, cyclic compounds including steroids, sterols, sterols glycosides and sterols esters are also present [18]. It is interesting to know that [14,16] - hentriacontanedione is the second most abundant (875mg/Kg fiber) single compound found in wheat straw wax, while octacosanol is the most abundant (1392mg/Kg fiber) single compound in this wax [18]. Sin reported the extraction and characterization of wheat straw wax using organic solvents and scCO2 [18].
Since wheat straw wax contains high amounts of β-diketone, this compound can be easily purified and used as a bio based chemical in metal chelation and many other applications Asemave [5], Asemave et al. [4]. Reported the extraction of lipophilic β-diketone from wheat straw wax using cuprous acetate and petroleum ether (333-353 K) with some modification from the previous report by Horn et al. [21]. Similar methodologies for isolating long chain β-diketones from plant waxes have previously been carried out, whereby petroleum ether (typically a C5 and C6 alkane mixture) or hexane were the solvents of choice [22]. However, these solvents are non-renewable and have a number of toxicological and safety issues associated with them. Hexane is an extremely flammable, forms explosive mixture with air and was found to be a neurotoxin, having detrimental effects on the nervous system [23]. Furthermore, hexane has been listed as a hazardous air pollutant by the Environment Protection Agency (EPA) in the Clean Air Act (1990) [24]. Therefore, substituting these hazardous, non-renewable solvents with greener solvents is vital in order to move towards a greener, more sustainable process. Cyclohexane, p-cymene, 2,2,5,5-tetramethylTHF (TMTHF) and cyclopentyl methyl ether (CPME) have greener credentials of environmental health safety (EH) compared to most traditional solvents [28,29]. Hence, they were considered as alternatives for the purification of the Htd from wheat straw wax. In addition to green credentials, the ability of these alternative solvents to dissolve the wheat straw wax is a critical property, without which the purification would not be possible. Jessop reported that there is currently a growing interest in using greener solvents so as to reduce solvent-related environmental damage [30].
One key parameter here is finding a low/moderate boiling point green solvent in order to avoid energy-intensive processes such as distillation and hence reduce energy costs [30]. In addition, scCO2 is one such alternative that was considered [25]. scCO2 extraction is a green technique, in which the CO2 is abundantly obtained as a co-product from fermentation, production of bioethanol, production of ammonia and the generation of hydrogen [19,26]. The scCO2 is nontoxic unlike most traditional volatile organic solvents used in extraction of plant waxes. In addition, the density of scCO2 has a crucial effect on the amount of lipids that is extracted from biomass. The highest scCO2 density is achieved at low temperature and high pressure [31]; with the latter in particular taking pre-eminence in scCO2 extraction. Moreso, it is possible to carry out fractionation of crude products with scCO2 using fractional separators found in series [27]. Each fractional separator is set at a different pressure and temperature which therefore causes a change in the density of CO2. Lipid molecules that are only soluble at a particular density range will crash out when entering a fractional separator having a different CO2 density, while those molecules that are still soluble will flow into the subsequent separator. This leads to wax products of higher value and could make isolation of certain compounds, such as β-diketones, more straight forward. Matricardi et al. [32] showed that for an effective fractionation, waxes are often adsorbed onto a solid support. This is done in order to decrease the particle sizes and give larger amounts of surface area to facilitate diffusion as well as an increased number of ruptured cells resulting into higher fractional yields. Also the presence of solutes in the supercritical phase act as a co-solvent which significantly enhances the solubilising ability of scCO2, a phenomenon referred to as the entrainer effect as previously described by Dobbs and Johnston [33]. No literature has been found looking into using greener alternative solvents in the purification of long chain saturated β-diketone. Therefore, this paper investigates the purification of the Htd using greener alternative solvents to petroleum ether and hexane; scCO2, p-cymene, CPME, TMTHF and cyclohexane.
Dichloromethane and cyclohexane were purchased from Fisher Scientific UK Limited. Wheat straw wax (was supplied by Green Chemistry Centre of Excellence, University of York), and carbon dioxide of > 99.99% purity (purchased from BOC Group). Cyclopentyl methyl ether, 2,2,5,5-tetramethylTHF, tetradecane, p-cymene were purchased from Sigma- Aldrich (analytical grade>99% purity). The instrument used were; GC-MS (Perkin Elmer Clarus 500 GC coupled with a Clarus 500 quadrupole mass spectrometer), GC-FID Agilent 7820A (Agilent 6890N, Hewlett Packard HP6890; column name, RXI-5HT; column diameter, 30 m x 0.25 mm x 0.25 μm and column maximum temperature of 673 K), FTIR Bruker Vertex 70, 1H-NMR and 13C-NMR (JNM - ECS 400 of field strength, 400 MHz for 1H and 100 MHz for 13C), MDSC Q 2000, LC - Agilent 1260 Infinity and MS - Bruker micrOTOF time of flight MS.
Purification of Htd using Cyclohexane, p-cymene, TMTHF or CPME with cuprous acetate: The isolation of the Htd was carried out as previously reported by Horn et al. [23]. With some slight modifications. A typical extraction procedure is as follows: About 0.1 g of the wheat straw wax was thoroughly crushed with spatula in a 250mL beaker and dissolved with 20mL each of cyclohexane, p-cymene, TMTHF or CPME under magnetic stirring at room temperature. This mixture was filtered after standing for 30 min and the filtrate transferred to 250mL separating flask, followed by addition of 20mL hot excess saturated aqueous Cu(OAc). The resulting mixture was shaken intermittently for 10 min and allowed to stand for 1h. The organic phase (upper layer) became green, while the aqueous phase (lower layer) was light-bluish with a dark- bluish Cu-diketone in between the two phases. The system was heated with heat-gun to afford proper separation of the organic and aqueous phase. The aqueous layer was removed while the organic layer was collected and kept for 2h for the complete crashing of the Cu-diketone. The precipitate was then filtered and the crude Cu-diketone washed with 1mL of cyclohexane, p-cymene, TMTHF or CPME respectively. The copper salt was re- dissolved in about 4mL of the hot cyclohexane, p-cymene, TMTHF or CPME and transferred into the 50mL separating flask follow by addition of 0.2mL concentrated hydrochloric acid. The separating flask and its contents was thoroughly shaken intermittently for 10 min to strip out the Cu(II) ions from the lipophilic β-diketone in the organic phase (Figure 1). The organic layer was washed with 10mL deionised distilled water stepwise. The two layers were separated and the pale yellow product (Htd) was recovered from the organic layer by removing the organic solvent under in vacuo. The excess aqueous cuprous acetate was recovered and re-used. 1H-NMR (400 MHz CDCl3) δppm 0.79 - 0.92 (m, 9H), 1.24 (s, 60H), 1.41 (s, 3H), 1.37 (s, 4H), 1.49 - 1.75 (m, 16H), 2.22 - 2.31 (m, 5H), 2.48 (t, j = 732 Hz, 1H), 3.53 (s, 1H), 5.46 (s, 1H).13C-NMR (101MHz, CDCl3) δ ppm 14.19 (1 C, s), 22.77 (1 C, s), 25.83 (1 C, s), 29.32 (1 C, s), 29.44 (1 C, s), 29.55 (1 C, s), 29.69 (1 C, s), 29.73 (1 C, s), 29.76 (1 C, s), 32.00 (1 C, s), 38.50 (1 C, s), 99.13 (1 C, s), 123.71 (1 C, s), 193.28 (1 C, s), 194.66 (1 C, s). FTIR (cm-1) - 2955, 2916, 2849, 1639, 1453, 1419, 1375, 1139, 907, 786, 722, 721, 631. GC-MS molecular ion; 464m/z; Yield 18±5wt%. Melting point, 53.9 OC; ESI-MS(+), 463.4506m/z, 503.4437 m/z, 549.4853m/z. The isolated product is as presented in Equation.
The scCO2 fractionation of wheat straw wax for Htd: The scCO2 fractionations of the wheat straw wax were carried out using Thar SFE-500 scCO2 extractor with 99.99% pure CO2. About 1g of wheat straw wax was crushed and dissolved with about 500mL methylene chloride (DCM) in 1000mL beaker, followed by addition of about 200g of celite, silica or 400g alumina adsorbent. The adsorbent was thoroughly mixed in the wax solution. Thereafter, the solvent was then completely removed in vacuo affording a dried uniform solid mixture of the wax- adsorbent. This solid mixture above was transferred into the 500mL extracting vessel for the scCO2 fractionation.
The fractionation of the wax was carried out sequentially by pre-setting the desired temperatures and pressures of the scCO2 separators [17,29,40]. An internal pump was used in order to obtain the required pressure. The system was run in dynamic mode, in which the scCO2 via the wax lipids was allowed to flow into the collection vessel. A flow rate of 40g/min of scCO2 was applied and the extraction was carried out for 40 min at 313 K and applying pressures of 75 bar, 100 bar, 200 bar, 300 bar and 400 bar. Then fractional lipids were collected by rinsing each separator vessel twice with approximately 100mL of DCM. When the extraction was terminated, depressurisation of the system was carried out over a period of 4h. The solvent in the fractions collected was removed under vacuum and the mass of extract determined. The wax: adsorbent residue in the extractor was removed and a brush was used to clean the extraction vessel.
GC-FID calibration procedure for Htd quantification: Accurately weighed samples of 0.3mg, 0.7mg, 4.1mg, 7.8mg and 16.7mg of Htd were each dissolved with 1mL of cyclohexane. Then 10μL, 2μL, 2μL, 2μL and 2μL of tetradecane (standard) were injected into the above Htd solutions respectively. GC-FID analysis of these samples was performed using GC-FIDS Agilent 7820A (Agilent 6890N, Hewlett Packard HP6890). The relative response of the Htd and tetradecane were obtained from their peak areas. Thereafter, the plot of relative response (peak area) against relative mass of Htd was made to obtain the response factor (Rf). The calibration for Htd with tetradecane as standard showed a linear response as described in Figure 2. The basis for this calibration is mathematically expressed in Equation 1. This response factor was used to determine the amount of the Htd in the wheat straw wax fractions collected from the scCO2 fractionation.
MassHtd/MassStd=Rf X AreaHtd/AreaStd
Equation 2: Mass and Area response ratios for the GC-FID calibration
Note: Rf=Response factor, GC peaks areas of the Htd and tetradecane are; AreaHtd and AreaStd respectively; and MassHtd and MassStd =Mass of the Htd and tetradecane
The isolation of the Htd from wheat straw wax was effectively achieved with CPME, TMTHF, p-cymene and cyclohexane with cuprous acetate. The recovered yields are presented in Table 1. The FTIR, NMR, GC-MS and ESI-MS were all the same as being previously reported by Asemave et al [4]. When the lipophilic β-diketone was extracted with petroleum ether; and hence the compound isolated is 14,16-hentriacontanedione. The removal of p-cymene (boiling point of 450K) from the product was carried out at substantially reduced pressure and 373K. This is very energy intensive and would not be viable from an economical point of view, and also resulted in residual p-cymene being left in the final isolated product. One possible way to reduce traces of the p-cymene in the product is to dissolve the copper-diketone complex in a more volatile green solvent prior to the decomposition of the complex with HCl to obtain the free Htd. Fortunately, CPME and TMTHF were readily removed at 333K with reduced pressures of about 50-60mbar. Moreso, the results implied that TMTHF produced the highest yield, whereas cyclohexane gave the lowest yield of Htd as presented in Table 1. However, CPME, TMTHF and cyclohexane could be effectively used as greener alternatives to petroleum ether (333-353 K) for the purification of the Htd.
Figure 3: Properties of the alternative solvents used in the purification of Htd from wheat straw wax in comparison to petroleum ether and hexane [28].
The properties of these alternatives solvents used for the isolation of Htd are given in Figure 3. Each solvent is given a score from 1 (red, bad) to 10 (green, good) to express their properties as compared to the traditionally used petroleum ether and hexane in the isolation of Htd. This guide has been previously reported by Henderson et al. [28], Alder et al. [33]. Some other advantages of these alternative solvents used for isolation of Htd like TMTHF and p-cymene is that they are bio derived. TMTHF can be produced by the mycelium of tuber borchii [34]. In addition, Green Chemistry Centre of Excellence (University of York) has developed a method for synthesis of bio-based TMTHF with an intention of replacing toluene with it. On the other hand, p-cymene can be easily derived quantitatively from limonene through sequential isomerisation and dehydrogenation [35]. Limonene in turn is available in large quantities from citrus waste [35]. Although the feedstock is limited by the geographical location and seasonality of the crop, fortunately extracting limonene from citrus rind does not compete with food production [35]. Limonene itself is a suitable bio-solvent that is able to replace non-polar petroleum-based solvents CPME has benefit of moderate boiling point (379 K) with better properties such as low formation of peroxides [37]. Low solubility in water, relatively stable under acidic and basic conditions [37].
The amount of Htd was evaluated from each scCO2 fractional extracts and the results are as found in Table 2. Furthermore, the classes of compounds present in the wheat straw wax were pre viously been identified as; ketones, fatty acids, alkanes, alcohol, aldehydes, β-diketones, sterols and wax esters by Sin [19]. The GC-FID chromatogram in Figure 1 explains that. Thus, this information was valuable in establishing the composition of the different scCO2 fractional extracts. The scCO2 fractional masses using celite were; 78.30mg, 338.90mg, 115.20mg, 22.90mg and 11.70mg at 75 bar, 100 bar, 200 bar, 300 bar and 400 bar respectively as given in Table 2. Therefore, the highest fractional mass, 338.90mg contained 171.36mg Htd (i.e. about 17% yield of Htd) at 100 bar. And the fraction at 400 bar shows smallest amount of the Htd, 0.80mg. The amount of the Htd was proportional to the fractional mass. Therefore, in our hands, 100 bar, using celite is at least appropriate for the purification of Htd. In addition to the presence of Htd, at a low pressure of 75 bar, more fatty acids and alkanes were detected than at higher pressures (100-400 bar), which is consistent with previous observations by Deswarte et al. [39], Hunt et al. [31], Cheung [39]. Whereas more waxy esters, and fatty alcohols were also found at higher pressures (100-400 bar) than at 75 bar. Hunt et al. observed that in the liquid scCO2 extraction of lipids from C4 biomass and fractionation of sugarcane bagasse, there were larger concentrations of wax esters at higher pressures (250 bar and 150 bar) than at lower pressures (150 bar and 1 bar); while at lower pressures higher concentrations of fatty acids were found [31]. Furthermore, Deswarte et al. [17] fractionated wheat straw using liquid scCO2 and observed that at relatively low pressures, the extract contains a high amount of alkanes whereas at higher pressures the extracts contain higher quantities of fatty alcohols.
For the silica adsorbent, smallest amount of the Htd, 0.94mg was found at 75 bar, while highest quantity of the Htd, 81.42mg (representing about 8% Htd) was obtained at 200 bar follow by 36.57mg Htd (i.e. 4% Htd) at 300 bar. The amount of the Htd was not proportional to the fractional mass. However, doing the sequential fractionation of the wheat straw wax for longer time of 3h at 75 bar, 313K in the presence of silica would leave behind a fraction with concentrated Htd. In addition, the sequential fractionations from 1g wax: 200g silica at 200 bar and 300 bar, 313K for 40 min has a reasonable selectivity for the Htd based on the compositional evaluation from the GC chromatograms. Moreso, Table 2 shows that these fractions contained higher amount of Htd than the fractions at 75, 100 and 400 bar. In addition to the Htd, there were higher compositions of alkanes, fatty acids, aldehydes and ketones at pressures of 100 and 75 bars than pressures of 200, 300 and 400 bar. Alumina adsorbent was also tested for the sequential scCO2 fractionation of the wax. 400g of the alumina was used with 1g of the wax instead of 200g in order to sufficiently load the extractor. The sequential extraction of this wax with alumina adsorbent shows that the Htd was not detected in fractions at 75 and 100 bar, while fractions 200, 300 and 400 bar contain the Htd with other components. Therefore, 0.17mg, 0.32 mg and 0.16mg of Htd were detected in the fractions at 200, 300 and 400 bar respectively (Figure 4). In which case, the fractionation using the alumina adsorbent was not effective because the % recovery of Htd in either of the fraction was <0.1%. Moreover, GC-FID chromatogram revealed that, the compositions of the fractions contained similar components. Hence, there was no selectivity for Htd with alumina.
Summarily, fractions with higher amount of the Htd were obtained at 100 bar, 171.36mg for celite adsorbent; 200 bar, 81.42mg for silica adsorbent and 300 bar, 0.32mg for alumina adsorbent. The observed total fractional mass in each case (567.0mg for celite, 449.3mg for silica and 39.7mg for alumina) were smaller than the 1g wax (initial feed). Since the time allotted for each fractionation was only 40 min, a total of 3h 20 min was taken to collect all the five fractions. Increasing the amount of time for the extraction at each pressure would enhance the total mass recovery. A total of 21.10wt%, 16.04wt% and 0.10wt% recovery of the Htd were found using celite, silica and alumina adsorbents respectively per gram of the wax. However, there were fractional masses with silica that produced best selectivity for Htd at 200 bar and 300 bar. Therefore, the order of effectiveness for purification of Htd with these adsorbents was silica >celite> alumina. The fractionation of the wax using the silica adsorbent appeared more promising in terms of selectivity towards the Htd. However, further optimization studies should be investigated about temperature, pressure and wax: silica ratio to further improve the scCO2 fractionation process for Htd.
Summarily, fractions with higher amount of the Htd were obtained at 100 bar, 171.36mg for celite adsorbent; 200 bar, 81.42mg for silica adsorbent and 300 bar, 0.32 mg for alumina adsorbent. The observed total fractional mass in each case (567.0mg for celite, 449.3 mg for silica and 39.7mg for alumina) were smaller than the 1g wax (initial feed). Since the time allotted for each fractionation was only 40 min, a total of 3h 20 min was taken to collect all the five fractions. Increasing the amount of time for the extraction at each pressure would enhance the total mass recovery. A total of 21.10wt%, 16.04wt% and 0.10wt% recovery of the Htd were found using celite, silica and alumina adsorbents respectively per gram of the wax. However, there were fractional masses with silica that produced best selectivity for Htd at 200 bar and 300 bar. Therefore, the order of effectiveness for purification of Htd with these adsorbents was silica >celite> alumina. The fractionation of the wax using the silica adsorbent appeared more promising in terms of selectivity towards the Htd. However, further optimization studies should be investigated about temperature, pressure and wax: silica ratio to further improve the scCO2 fractionation process for Htd.
This research demonstrated that Htd was effectively isolated from wheat straw wax with Cu(OAc)2 using alternative greener solvents to hexane and petroleum ether (333-353 K); cyclohexane, TMTHF and CPME. In which case TMTHF gave the highest yield of 21.40wt% of Htd among these alternative solvents tested. P-cymene could also be used but it has a higher boiling point which will consume more energy during distillation to separate p-cymene from the Htd. scCO2 was also tested for fractionation of the wheat straw wax in order to obtain the Htd using different adsorbents (celite, silica and alumina) and varying pressures. Sequential scCO2 fractionation of 1g wax: 200g silica at 200 bar, 300 bar and 313K for 40 min was found to give at least better selectivity for the Htd than with alumina and celite adsorbents under the same condition. However, the scCO2 fractionation of the wax for Htd needs to be optimized especially with the adsorbent silica.
We thank the TET Fund Nigeria for the grant and Green Chemistry Centre of Excellence, University of York where this research was carried out.
Bio chemistry
University of Texas Medical Branch, USADepartment of Criminal Justice
Liberty University, USADepartment of Psychiatry
University of Kentucky, USADepartment of Medicine
Gally International Biomedical Research & Consulting LLC, USADepartment of Urbanisation and Agricultural
Montreal university, USAOral & Maxillofacial Pathology
New York University, USAGastroenterology and Hepatology
University of Alabama, UKDepartment of Medicine
Universities of Bradford, UKOncology
Circulogene Theranostics, EnglandRadiation Chemistry
National University of Mexico, USAAnalytical Chemistry
Wentworth Institute of Technology, USAMinimally Invasive Surgery
Mercer University school of Medicine, USAPediatric Dentistry
University of Athens , GreeceThe annual scholar awards from Lupine Publishers honor a selected number Read More...