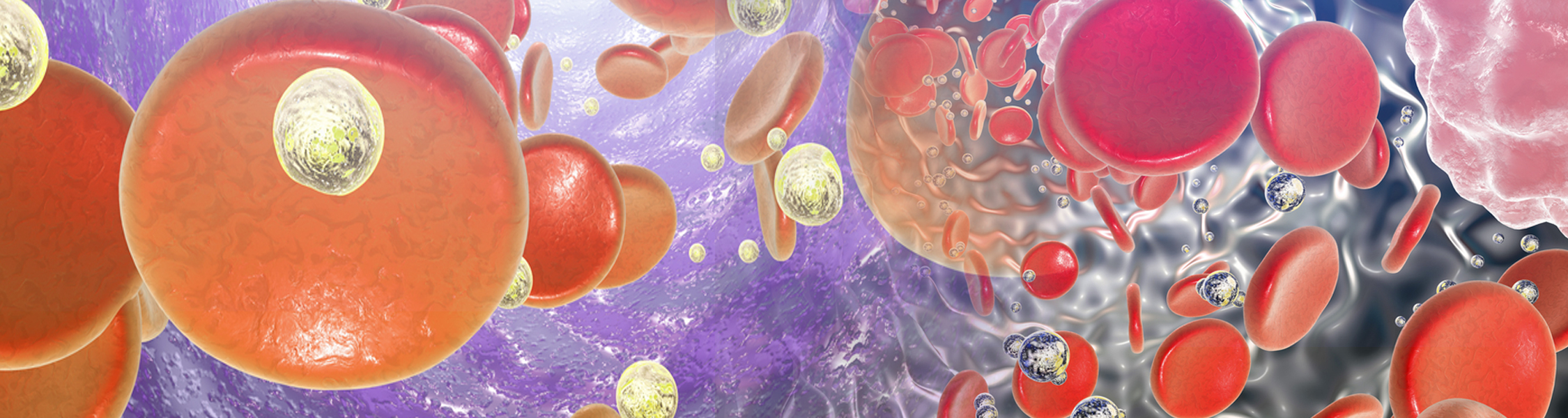
Seyfettin Dalgiç* and Göze Gökçe Arikan
Received: April 1, 2022 Published: April 14, 2022
Corresponding author: Seyfettin Dalgiç, Trakya University, Science Faculty, Department of Physics, Edirne, Turkey
DOI: 10.32474/JBRS.2022.01.000120
Today, mainly oil, coal, and natural gas are used for energy in many countries. It is possible that these resources will be limited and will run out in the future. These resources, fossil fuels, are not renewable. Renewable energy is commonly described as energy sources that can be provided mostly above ground and continuously renewed without any production process. In recent years, the development and use of renewable energy sources have become essential. Choosing the materials used in this energy from nanomaterials would be beneficial. Therefore, in this study, nanomaterials with three different structures, such as body-centred cubic (bcc), face-centred cubic (fcc), and hexagonal closed-pack (hcp), were selected. Thermal conductivity and thermal expansion calculations have been made for these materials. Analyses completed with intensive computer use and their results are given comparatively. In the calculations obtained, the effect of the selected parameters has been investigated and nanomaterials that could be used in renewable energy have been discussed.
Keywords: Renewable Energy; Nano Materials; Thermal Conductivity;Thermal Expansion
Nanoscience and nanotechnology are among the most used terms in the modern scientific and technological literature. The idea of nanotechnology first emerged on December 29, 1959, in the famous speech “There’s a lot of ground at the bottom” given by physicist Richard Feynman at Caltech, USA[1]. The term nanotechnology was originally defined by Taniguchi Norio in 1974 as follows. “Nano technology mainly consists of the separation, assembly and deformation of materials by an atom or a molecule” [2]. Nanotechnology and nanoscience began with two major developments in the early 1980s. The first of these is the increase in computational power. The second is advances in material modeling. These areas are now growing very rapidly.
Nanomaterials cross the border between nanoscience and nanotechnology and connect these two fields. In general, nanomaterials relate to sizes 100 nanometers or less in at least one dimension. The material properties of nanostructures differ from bulk structu res due to the high surface area to volume ratio and the emergence of possible quantum effects at the nanoscale. The study of the effects of size and shape on material properties has received great attention due to their scientific and industrial importance.
It is believed that nanomaterials will be the soul of the next technological development in all fields of science. Nanomaterials differ significantly from bulk structure, especially due to their small size. Various physical properties such as hardness, melting temperature, elastic modulus, and electronic structure can depend on particle size. It has been reported that nanomaterials, nanoparticles, nanowires and nanotubes exhibit distinctly different physical, chemical and mechanical properties from their corresponding bulk structure numbers [3].
In nanomaterial, free surface atoms are different from bulk atoms and are located on the surface. Therefore, the energy associated with these atoms will be different from the energy of the bulk structure. The extra energy shared with surface atoms is called surface energy. Surface free energy is typically neglected in conventional continuum mechanics. Because it is associated with only a few layers of atoms near the surface, it is much smaller than the ratio of the volume occupied by the surface atoms and the total volume of the material of interest. Recently, nanomaterials, which form the basis of nanoscience and nanotechnology, have been widely included in the scientific literature. It has also become a much studied interdisciplinary research and development area. Especially with the increasing interest in renewable energies, the importance of these materials has also increased. Working with nanomaterials is exciting. Because they exhibit strong shape and size effects that cannot be explained by typical theories. Therefore, studies on this subject will continue to increase day by day[4].
This article is planned as follows. Information about nanomaterials, nanoscience and nanotechnology was given. An introduction was created by giving the relationship between renewable energy and nanomaterials. Theoretical calculations of thermal conductivity and thermal expansion of nanomaterials are discussed. These properties of selected nanomaterials were calculated by intensive computer calculations. In the discussion and results section, the results are presented in tables and graphs. The comment of article was made In the conclusion.
Thermal conductivity and thermal expansion calculations in nanomaterials have an important place in examining the size and shape effects of the material. These sizes are given depending on either the temperature or the material shape. It is in the form of thermal conductivity of nanomaterials.
Carriage return, kp and kb are the thermal conductivity of the nanoparticle and the bulk material, respectively. The N/n value takes 4d/D, 8d/3l and 4d/3h for spherical nanosolids, nanowires and nanofilms, respectively. Also, d is the diameter of the nanosolid and D is the diameter of the spherical nanosolid. l and h are the diameter of the nanowire and the height of the nanofilm, respectively[ 5]. Although many experimental studies have been conducted to examine the effect of size and temperature on nanomaterials, theoretical studies are very few. Here, an equation of state (EoS) for nanomaterials is obtained under the influence of particle size and temperature by taking a simple theoretical approach to study the expansion behavior. This equation is used to calculate V/V0 for spherical nanosolids, nanowires, and free surface nanofilms. The expressions V/V0 for nanosolids, nanowires and nanofilms are given by the following equations, respectively.
where,
The volume dependence of the thermal expansion coefficient is given below.
The relationship between the bulk modulus and coefficient of thermal expansion of the Anderson Gruneisen parameter is given as follows.
In this work, firstly, based on surface effects, the thermal conductivity of some nanomaterials based on size dependent atomic structure is calculated. The second is to calculate the temperature- dependent changes of the nanomaterial volume ratios. Calculations were made for materials with 3 different structures. These are body-centered cubic (bcc) and face-centered cubic (fcc), and hexagonal closed-pack (hcp) respectively[7]. All input parameters used in the calculations are taken from A.C. Lawson[8]. Also these calculations have been made on nanosolids, nanowires and nanofilms. The interfacial volume ratio of nanocrystals is large. Although surface effects are normally insignificant for bulk materials, they have a very important place in them. Some nanoscale materials exhibit many interesting chemical and physical properties that cannot be observed in other materials. The bond and bond energy densities of such structures are important.
The input parameters of the nanomaterials with bcc structure are given in Table 1. By using the input parameters given in the table and equation 1b, thermal conductivities depending on size were calculated for Li, Na, Cr and Fe in bcc structure. The results obtained are shown in Figure 1. As can be seen from the figures, calculations were made separately for nanosolids, nanowires and nanofilms. In all materials, thermal conductivity is lowest in nanosolids, nanowires in the middle, and highest in nanofilms. In all materials, thermal conductivity is lowest in nanosolids, nanowires in the middle, and highest in nanofilms. The following comment can be made here. Since the particle size is in the form of nanosolid, nanowire and nanofilm, it gives appropriate results in thermal conductivity. While there is a rapid increase up to the values of D (0-16) au, the increase slows down and even becomes stable after 16 au. In addition, the nanosolid, nanowire and nanofilm states of these materials are given in Figure 2 comparatively. When the figure is looked carefully, it is seen that Na is at the bottom and Li is at the top for each case. Fe and Cr are in the middle. It was determined that this situation was caused by δT values. The input parameters of the nanomaterials with fcc structure are given in Table 2.
Figure 1: Thermal conductivity change depending on grain size for Li, Na, Cr and Fe (spherical nanosolid, nanowire and nanofilm) in bcc structure.
Figure 2: Comparison of the thermal conductivity for Li, Na, Cr and Fe in spherical nanosolid, nanowire and nanofilm.
With the input parameters given in the table, thermal conductivities were calculated for Al, Ca, Ag and Au in fcc structure depending on the size. Obtained results are shown in Figure 3. As can be seen from the figures, calculations were made separately for nanosolids, nanowires and nanofilms. Similar features in the bcc structure show themselves here as well. It has been observed that the thermal conductivity is lower in nanosolids. Depending on the particle size, it is in the order of nanosolid, nanowire and nanofilm. The nanosolid, nanowire and nanofilm states of these materials in fcc structure are given in Figure 4 comparatively. As can be seen from the figures, for each case, Ca is at the bottom and Al is at the top. Ag and Au are in the middle. It has been determined that this situation depends on the δT values. With these parameters, thermal conductivities were calculated for Mg, Co and Cd in the hcp structure depending on the size. Obtained results are shown in Figure 5. Depending on the particle size, thermal conductivity ranges become very evident in nanosolids, nanowires and nanofilms. This distinction continues after the au values of D (0-16). The nanosolid, nanowire and nanofilm states of these materials in this structure are given in Figure 6 comparatively. As can be seen from the figure, the values for each case are very close to each other, but Mg is at the bottom and Cd is at the top. Co is in the middle. Again, it was determined that this situation depends on the δT values.
Figure 3: Thermal conductivity change depending on grain size for Al, Ca, Au and Ag (spherical nanosolid, nanowire and nanofilm) in fcc structure.
Figure 4: Comparison of the thermal conductivity for Al, Ca, Au and Ag in spherical nanosolid, nanowire and nanofilm.
Figure 5: Thermal conductivity change depending on grain size for Mg, Co and Cd (spherical nanosolid, nanowire and nanofilm) in hcp structure.
Figure 6: Comparison of the thermal conductivity for Mg, Cö and Cd in spherical nanosolid, nanowire and nanofilm.
Figure 7: Temperature dependent comparison of V/V0 for Li, Na, Cr and Fe in bcc structure (spherical nanosolid, nanowire and nanofilm).
Figure 8: Temperature dependent comparison of V/V0 for Al, Ca, Ag and Au in fcc structure (spherical nanosolid, nanowire and nanofilm).
Figure 9: Temperature dependent comparison of V/V0 for Mg, Co and Cd in fcc structure (spherical nanosolid, nanowire and nanofilm).
Using the same table values, temperature-dependent calculations of volume ratios were made for all materials. In these calculations, it was seen that the graphs were quite similar to each other. Therefore, it was decided to compare all materials for nanosolid, nanowire and nanofilm. Comparison graphs are given in Figure 7 for bcc structure, Figure 8 for fcc structure and Figure 9 for hcp structure, respectively. When the figures are examined carefully, in the bcc structure, Na is at the top and Cr is at the bottom. Fe and Li are located in the middle. Na volume ratio is large, Cr volume ratio is small. These properties are the same in nanosolid, nanowire and nanofilm materials. It is concluded that the temperature dependence of the volume ratios does not change according to the material type. In the fcc structure, Al and Ca are at the top with almost the same value, Au at the bottom. Ag is located in the middle. Al and Ca volume ratio is large, Au volume ratio is small. Here, the situation is the same for nanosolid, nanowire and nanofilm materials. However, the temperature dependence of the volume ratios does not differ in all materials. In the hcp structure, Cd is at the top and Co is at the bottom. Mg is located in the middle. This is the case for nanosolid, nanowire and nanofilm materials. The temperature dependence of the volume ratios in the hcp structure is that it does not change according to the material type.
The size dependent thermal conductivity of nanomaterials has been calculated with a model based on Lindemann criteria and surface effects. In each of the bcc, fcc and hcp structures, nanosolid, nanowire and nanofilm ordering were found in the same way. Although these three types of materials are very close to each other in bcc and fcc structures after 16 au value of D, the difference between them is more evident in other structures. It is an important theoretical method to examine the thermal dependence of their thermal expansion at higher temperatures. In this case, the size dependence and surface effect of the nanomaterial should be considered. At the same time, the Thermodynamic Equation of State (EoS) analysis provides information on the thermal expansion of nanomaterials based on theoretical thermodynamic relationships. While the coefficient of thermal expansion increases with decrease in particle size, it can be observed that V/V0 increases with temperature increase in nanomaterials of different particle sizes. Depending on the particle size, thermal conductivity and thermal expansion values are important for designing and producing renewable energy materials. These calculations are necessary for material selection and efficiency.
Bio chemistry
University of Texas Medical Branch, USADepartment of Criminal Justice
Liberty University, USADepartment of Psychiatry
University of Kentucky, USADepartment of Medicine
Gally International Biomedical Research & Consulting LLC, USADepartment of Urbanisation and Agricultural
Montreal university, USAOral & Maxillofacial Pathology
New York University, USAGastroenterology and Hepatology
University of Alabama, UKDepartment of Medicine
Universities of Bradford, UKOncology
Circulogene Theranostics, EnglandRadiation Chemistry
National University of Mexico, USAAnalytical Chemistry
Wentworth Institute of Technology, USAMinimally Invasive Surgery
Mercer University school of Medicine, USAPediatric Dentistry
University of Athens , GreeceThe annual scholar awards from Lupine Publishers honor a selected number Read More...